Reactions of MoCl5 and MoO2Cl2 with Succinimide, 1, 4-Diaminobutane, 3-Methylpyridine, 1, 3-Diaminopropane, Pyrazole and 1- Methylpyrrolidine in Tetrahydrofuran
1Punjab Technical University, Kapurthala India.
2Department of Applied Chemistry, Giani Zail Singh Campus College of Engineering and Technology, Dabwali Road, MRSPTU Bathinda-151001 India.
Corresponding Author E-mail: gursharans82@gmail.com
DOI : http://dx.doi.org/10.13005/ojc/370306
Article Received on : 04-Jun-2021
Article Accepted on :
Article Published : 23 Jun 2021
It has been reported that molybdenum may extract oxygen from oxygen containing ligands. Oxo complexes of above bases with transition metals show numerous applications and are biologically active. So to study the biological activity of molybdenum complexes and to study oxo abstraction reactions by molybdenum, reactions of succinimide/1, 4-diaminobutane/3-methylpyridine/1, 3-diaminopropane/pyrazole/1-methylpyrrolidine with MoCl5/MoO2Cl2 have been carried out, in THF medium using equimolar/bimolar quantities of the ligand, at normal temperature. The products thus obtained are:
Mo2O3Cl5(C4H5NO2)2(C4H8O)2, [1]; Mo2O2Cl2(C4H5NO2)2(C4H8O)2, [2]; MoO2Cl2(H2NCH2CH2CH2CH2NH2)2, [3]; Mo3Cl8(C6H7N)4(C4H8O)2, [4]; Mo3Cl6(C6H7N)6(C4H8O)6, [5]; MoO2Cl3(H2NCH2CH2CH2NH2)2, [6]; Mo2O4Cl4(C3H4N2)4, [7] and Mo2O6Cl8(C5H11N)4, [8]. There is oxygen abstraction by molybdenum during the reaction from the oxygen containing solvent THF.
Formulations of these compounds were made and their properties were studied with FTIR(transmission mode), 1H NMR/13C NMR, microbiological studies, elemental analysis(Mo, Cl, C, H, N) and LC-MS. All preparations, separations and isolations were executed in vacuum line and inert atmosphere (dry nitrogen) to eliminate any oxidation/hydrolysis of products by air/moisture. The formulations proposed have been supported by the above characterization studies.
1, 3-diaminopropane; 1, 4-diaminobutane; 1-methylpyrrolidine; 3-methylpyridine; pyrazole
Download this article as:
Copy the following to cite this article: Kumar R, Singh G. Reactions of MoCl5 and MoO2Cl2 with Succinimide, 1, 4-Diaminobutane, 3-Methylpyridine, 1, 3-Diaminopropane, Pyrazole and 1-Methylpyrrolidine in Tetrahydrofuran. Orient J Chem 2021;37(3). |
Copy the following to cite this URL: Kumar R, Singh G. Reactions of MoCl5 and MoO2Cl2 with Succinimide, 1, 4-Diaminobutane, 3-Methylpyridine, 1, 3-Diaminopropane, Pyrazole and 1-Methylpyrrolidine in Tetrahydrofuran. Orient J Chem 2021;37(3). Available from: https://bit.ly/3xBHGl4 |
Introduction
Succinimide
Succinimides1 are involved in various biological applications. Succinimide is a constituent of various biologically active compounds having significance as: CNS depressant2, hypotensive3, antitumour4, cytostatic5, bacteriostatic6, nerve conduction blocking7, muscle relaxant8, anorectic9, antibacterial10, analgesic11, anti-convulsant12, anti-tubercular13, antispasmodic14 and antifungal15.
Succinimide can form complex through oxygen atom. Deprotonated succinimide (on removal of hydrogen from nitrogen)can form complex through the nitrogen atom. In deprotonated succinimide, the CN bond length lies between single and double bond, because the new free electron pair of nitrogen gets delocalized and is spread on both CN bonds due to conjugation between lone pair of nitrogen and π-electrons of C=O bonds16. This conjugation reduces chances of availability of this lone pair for coordination. So coordination in succinimide through oxygen is more likely.
1, 4-Diaminobutane
1, 4-Diaminobutane17-19 has a variety of applications in agrochemicals, paint additives, pharmaceuticals, surfactants and micronutrients. It is used as starting material in some biological systems and amido-ureas. It is used in the preparation of nylon 46. It acts as corrosion inhibitor of mild steel in 1N H2SO4.
3-Methylpyridine
3-Methylpyridine20 has application in agrochemical industry as chlorpyrifos21. 3-Methylpyridine degrades/evaporates slowly from water samples as compared to 2-methylpyridine/4-methylpyridine22, 23. 3-Methylpyridine is precursor to prepare antidote for organophosphate poisoning24. It is used as waterproofing agent25 in textile industry.
1, 3-Diaminopropane
1, 3-Diaminopropane26 has a variety of industrial applications, such as, in epoxy resin and cross-linking agents. It is used as precursor for preparation of pharmaceuticals, organic chemicals and agrochemicals. It is used27 in the synthesis of heterocycles used in coordination complexes and textile finishing. It is a potential inhibitor28, 29 against neoplasia and ornithine decarboxylase enzyme protein on rat urinary bladder carcinogenesis.
Pyrazole
Pyrazoles as well as their derivatives have a lot of biological properties, like antipshycotic30-32,antimicrobial33, anticonvulsant34, antitumor35, anti-cyclooxygenase36, analgesic37, antitubercular38, antidiabetic39, antiinflammatory40, etc.
1-Methylpyrrolidine
1-Methylpyrrolidine41 has numerous applications in agrochemicals, pharmaceuticals, colourants, organic synthesis, photographic chemicals, plasticizers, emulsifiers, rubber chemicals, curing agent for epoxy resins, corrosion inhibitors, etc. It is used in PU manufacture as a catalyst. In cosmetics,it acts as a powerful surfactant.It is present in cigarette smoke. It is part of cefepime42: broad-spectrum cephalosporin antibiotic capable to treat bacteria causing pneumonia, infections of the skin and urinary tract.
Transition metals complexes on coordination withligandsundergo deep change in physiological properties of the metals and ligands. Desired properties27, 43 on transition metals for particular applications can be incorporated by coordination of metals with certain ligands. It involves modification of properties, like stability of oxidation states, electrophilic/nucleophilic properties and solvophilicity of the metal ions. We have to choose a suitable metal and the ligand after various trials. On coordination, metal and ligand properties undergo a desired change. Many drugs containing metal chelates have higher biological activity than the uncoordinated ligands themselves44-49.
Aim of Investigation
MoCl5 reacts with a variety of bases. The author has investigated50-55 the reactions of MoCl5 with 4-phenylimidazole-2-thiol, aromatic azoles, imides, diaminoalkanes, alkylpyridines, 2-thiazoline-2-thiol, thiols and mercaptopyridine-N-oxide sodium.
MoO2Cl2 reacts with various bases. The author has investigated50-58 the reactions of MoO2Cl2 with aromatic azoles, alkanediols, diaminoalkanes, imides, amides, thioamides, purine and thiols.
It has been reported that molybdenum may extract59, 60 oxygen from oxygen containing ligands. Oxo complexes of above bases with transition metals show numerous applications and are biologically active. So to study the biological activity of molybdenum complexes and to study oxo abstraction reactions by molybdenum, reactions of succinimide/1, 4-diaminobutane/3-methylpyridine/1, 3-diaminopropane/pyrazole/1-methylpyrrolidine with MoCl5/MoO2Cl2 have been carried out. Formulations of these compounds were made and their properties were studied with FTIR, 1H NMR/13C NMR, microbiological studies, elemental analysis and LC-MS.
Materials and Methods
Succinimide, 1, 4-diaminobutane, 3-methylpyridine, 1, 3-diaminopropane, pyrazole, 1-methylpyrrolidine, MoCl5 and MoO2Cl2were procured from Sigma-Aldrich.
The reactants and products are sensitive to air/moisture, so all preparations, separations and isolations were executed in vacuum line and dry atmosphere (dry nitrogen) to eliminate any oxidation/hydrolysis of reactants/products by air/moisture.
Ligand solution in dry THF was dropped from dropping funnel with continuous agitation to MoCl5/MoO2Cl2 solution in THF taken in 100 ml round bottom flask. The reaction was carried out for about 7 h. The products were isolated after filtration through filtration unit fitted with G-4 sintered glass crucible.
Oxinate gravimetric method 61 was used formolybdenum estimation. Mixture of sodium carbonate and sodium peroxide was fused with a known weight of the sample by using nickel crucible. Contents were fused in muffle furnace for 1 hr. at 400º C. Fused mixture was extracted with distilled water and content was filtered through fine filter paper. Discarded the residue and only filtrate was retained. Added methyl red indicator to the filtrate. 2 N sulphuric acid was added to it dropwise to make it acidic. Added 2N ammonium acetate solution dropwise until colour of solution became faint. Solution was heated to boiling. Added 3% oxine solution (in glacial acetic acid) dropwise until yellow precipitates obtained. Boiled gently with stirring until the precipitation was complete. Filtered the yellow precipitate with G-4 sintered glass crucible Precipitate were washed with hot water, dried at 130-140° C and weighed as MoO2(C9H6NO)2.
Estimation of chlorine was carried out gravimetrically as silver chloride61. A known weight of the sample was taken in distilled water. Added 10-12 pallets of sodium hydroxide in it. Content was boiled, cooled and filtered through a fine filter paper. Acidified the solution with dilute nitric acid. Added excess of N/10 aqueous solution of AgNO3 until white precipitate of AgCl were obtained. Boiled the solution until precipitation and coagulation were complete. Filtered the precipitate through G-4 sintered glass crucible, washed with acetone, dried at 130-140° C and weighed as AgCl.
Carbon, hydrogen and nitrogen were estimated by Thermo Finnigan Elemental Analyser. Perkin-Elmer 400 FTIR Spectrometer was used for obtaining infrared spectra (transmission mode). Multinuclear Brucker Avance-II 400 NMR spectrometer was used for recording1H/13C NMR in DMSO-d6 solvent.LC-MS spectra in the range 0-1100 m/z have been attained. These studies were executed in SAIF at P. U. Chandigarh.
Molybdenum compounds prepared were tested using agar well diffusion assay methodfor their antibacterial and antifungal potential on the strains: Staphylococcus aureus (gram positive bacteria) (MTCC-737), E. coli (gram negative bacteria) (MTCC-1687), Candida albicans (fungus) (MTCC-227) and Aspergillus niger (fungus) (MTCC-282).Standard drugs amoxicillin and ketoconazole were used for bacteria and virus, respectively as reference. Cultures of MTCC (The Microbial Type Culture Collection and Gene Bank, Chandigarh, India) were used. Drug testing at ISF Analytical Laboratory (ISF College of Pharmacy), Ferozepur Road, Moga, Punjab (India) was carried out.R (Residue)/ F (Filtrate) refer to product source.
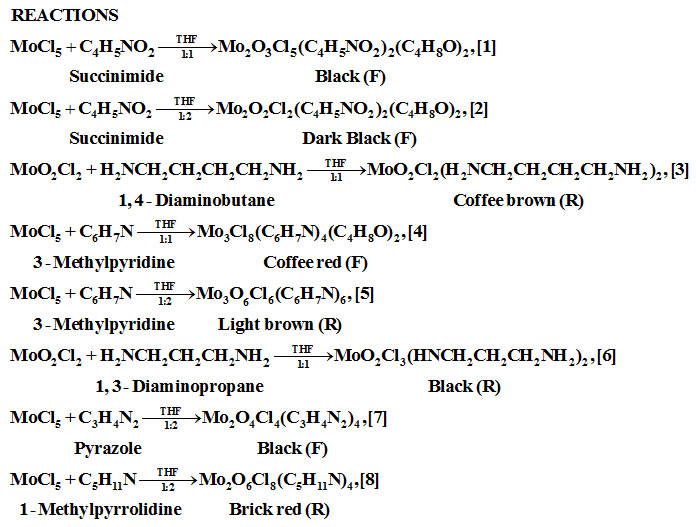
Results and Discussions
Elemental Estimation
Percentage of the observed (theoretical) values of the elements has been depicted in Table-1.
Table 1: Elemental Estimation
Compounds |
Cl |
Mo |
H |
C |
N |
Mo2O3Cl5(C4H5NO2)2(C4H8O)2, [1] (Black/759.5) |
22.87 (23.37) |
24.73 (25.27) |
03.23 (03.42) |
24.67 (25.27) |
04.13 (03.68) |
Mo2O2Cl2(C4H5NO2)2(C4H8O)2,[2] (Dark black/637.0) |
11.55 (11.14) |
29.42 (30.14) |
03.68 (04.08) |
29.87 (30.14) |
04.74 (04.39) |
Mo2O2Cl2(H2NCH2CH2CH2CH2NH2)2, [3] (Coffee brown/375.0) |
18.23 (18.93) |
24.73 (25.60) |
06.48 (06.40) |
24.79 (25.60) |
14.27 (14.93) |
Mo3Cl8(C6H7N)4(C4H8O)2, [4] (Coffee red/1088.0) |
25.33 (26.10) |
25.69 (26.47) |
03.39 (04.04) |
34.67 (35.29) |
04.64 (05.14) |
Mo3O6Cl6(C6H7N)6, [5] (Light brown/1055.0) |
17.73 (18.44) |
24.69 (24.93) |
03.93 (03.63) |
36.78 (37.40) |
06.98 (07.27) |
MoO2Cl3(HNCH2CH2CH2NH2)2, [6] (Black/380.5) |
27.02 (27.98) |
24.67 (25.22) |
05.13 (04.73) |
17.98 (18.92) |
14.13 (14.71) |
Mo2O4Cl4(C3H4N2)4, [7] (Black/670.0) |
20.68 (21.19) |
27.93 (28.65) |
02.71 (02.38) |
20.98 (21.49) |
16.04(16.71) |
Mo2O6Cl8(C5H11N)4, [8] (Brick red/912.0) |
30.47 (31.14) |
20.28 (21.05) |
05.36 (04.82) |
27.11 (26.31) |
05.84 (06.14) |
FTIR Spectra
N-H stretching of succinimide62, 63havebeen notedat 3409 cm-1 & 3221 cm-1. Strong absorption at 3294 cm-1 indicates that [1] contains N-H group. Bands at 980 cm-1 and 919 cm-1 support the availability of cis-MoO22+ core64, 65in [1]. Occurrence of cis-MoO22+ core is due to oxo abstraction59, 60 by molybdenum from THF. There is some decrease in C=O sym and asym absorptions. There is decrease in C=O bond order, referring to the presence of O→Mo coordination16 in [1] (Table-2).
Strong absorption at 3433 cm-1 indicates that [2] contains N-H group. Bands at 984 cm-1 and 923 cm-1 support the availability of cis-MoO22+ core64, 65in [2]. Occurrence of cis-MoO22+ core is due to oxo abstraction59, 60 by molybdenum from THF. There is some decrease in C = O sym and asym absorptions. There is decrease in C = O bond order, referring to the presence of O → Mo coordination16 in [2] (Table-2).
Table 2: FTIR absorptions in cm−1
Assignments |
Succinimide62, 63 |
[1] |
[2] |
N-H str. |
3409 s b, 3221 s b |
3509.6 sh, 3381.5 sh, 3294.4 s, 3152.4 s, b |
3433.5 s b |
CH2 sym. str. |
2960, 2947 w |
2983.7 sh |
|
C = O sym., H – N – C in plane bending |
1774 m |
1776.9 m, 1756.0 m |
1772.8 w |
C = O asym., H – N – C in plane bending |
1710 vs, b |
1686.0 s |
1705.2 s, 1637.2 m |
CH2 sym. scissoring |
1430 m |
1415.1 sh |
|
CH2 asym. scissoring |
1401 m |
1399.1 sh |
1399.8 sh |
C – N – C asym. str., H – N – C in plane bending |
1347 s, 1337 |
1373.7 m, 1358.9 sh |
1373.3 w |
CH2 bending, ring in plane bending |
1298 s |
1297.9 s |
1298.1 w |
CH2 bending |
1240 |
1248.1 m |
1247.1 sh |
C – N – C asym. str., H – N – C in plane bending |
1189 s, |
1183.2 s |
1189.9 m |
C – C str., CNC sym. str. |
849 |
857.2 w |
|
CH2 bending, ring out of plane bending |
820 s |
817.1 s |
|
|
722.9 s |
725.1 m b |
|
OCN asym. out of plane bending |
632 m |
645.9 s |
642.4 m |
OCN sym. out of plane bending, CH2 bending |
539 w |
552.2 w |
563.2 m |
Mo-N str. |
|
418.0 sh |
|
Mo=O str. of cis-MoO22+ core64, 65 |
980.6 s, 919.1 w |
984.7 w, 923.5 sh |
N-H stretching of 1, 4-diaminobutane66 hasbeendetectedat 3345 cm-1 & 3278 cm-1. Strong N-H absorptions have been recorded at 3391 cm-1, 3077 cm-1 and 3010 cm-1 in [3] (Table-3). Terminal Mo=O stretching occurs67 at 990 cm-1 -1010 cm-1 in various inert solvents. A medium Mo=O stretching64, 67, 68 at 921 cm-1 conforms to the presence of terminal Mo=O group. There is a decline in Mo=O stretching to 921 cm-1 showing Mo coordination69 to 1, 4-diaminobutane through N atom, in a direction trans to Mo=O bond. Bending mode due to NH2 observed in 1, 4-diaminobutane66 at 1146 cm−1 is declined to 1116 cm−1, because of N→Mo coordination.
Table 3: FTIR absorptions in cm−1
Assignments |
1, 4-Diaminobutane66 |
[3] |
N-H str. |
3345, 3278 |
3391.2 s, 3077.1 s, 3010.1 s |
CH2 str. |
2961-2874 |
2881.1 sh |
NH2 bending |
1608 |
1614.2 s |
CH2 deformation (strong) |
1498, 1391, 1355, 1310 |
1519.3 m, 1470.7 m, 1448.1 s, 1403.3 w, 1344.4 w |
NH2 bending |
1146 |
1184.4 w, 1116.2 s |
C-N sym str. (weak) |
1071 |
1025.3 m |
CH2 deformation (medium) |
862, 736 |
817.1 s |
Mo-N (strong) |
498.3 m |
|
Terminal Mo=O64, 67, 68str. |
921.2 m |
3-methylpyridine70-73showsring C-H absorptions at 3060 cm-1 and 3032 cm-1. Strong bands at 3119 cm-1 and 3054 cm-1 have been noticed in [4]. Ring C=N stretching & ring C=N torsion wave numbers have increased and ring C-H bending mode wave numbers have declined due to Mo(dπ)→N(pπ) back bonding. A strong band at 989 cm-1 revealsthe presence of terminal Mo=O64, 67, 68group in [4] (Table-4).
Strong band at 3391 cm-1 has been noticed in [5]. Ring C=N stretching & ring C=N torsion wave numbers have increased and ring C-H bending mode wave numbers have declined due to Mo(dπ)→N(pπ) back bonding. A medium band at 989 cm-1 revealsthe presence of terminal Mo=O64, 67, 68group in [5] (Table-4). Occurrence of terminal Mo=O is due to oxo abstraction59, 60 by molybdenum from THF.
Table 4: FTIR absorptions in cm−1
Assignments |
3-Methylpyridine70-73 |
[4] |
[5] |
C-H ringstr. |
3060, 3032 |
3168.9 s, 3119.9 s, 3054.8 s |
3391.0 s b |
C-H methylstr. |
3002, 2958, 2928 |
2937.5 sh, 2866.8 sh |
|
ringstr. |
1600, 1578 |
1629.1 m, 1607.1 m, 1543.1 s |
1630.7 s, 1554.6 s |
Ring C-H bending |
1478 |
||
C-H methyl assym bending |
1458, 1452 |
1469.1 m |
1474.2 m |
Ring C-H bending |
1415 |
|
|
C-H methyl sym. bending |
1388 |
1387.1 w |
1386.2 w |
Ring C-H bending |
1361 |
1306.2 w |
|
Ring str. |
1250 |
1258.1 w |
1264.3 w |
C-C bond between ring and methyl str. |
1228 |
||
Ring C-H bending |
1190 |
1180.2 w |
1186.2 sh |
C-H methyl rocking |
1127, 1044 |
1116.9 s |
1119.2 w |
Ring out of plane bending |
1030 |
1044.1 sh, 1019.1 sh |
1048.5 w |
Ring C=N str. |
792 |
883.1 vs, 785.7 s, 738.7 m |
891.1 s, 788.1 s |
Ring C=N torsion |
710 |
722.7 m |
721.9 m |
Ring bending |
635, 540 |
676.6 s, 511.1 m |
678.0 s, 630.3 sh, 568.4 sh, 513.8 sh |
∂ C-C bond between ring and methyl |
458 |
463.1 m |
463.3 w |
Terminal Mo=O64, 67, 68 str. |
989.9 s |
949.9 m |
1, 3-Diaminopropane74 shows N-H absorption bands in the range 3052-3351 cm-1. Absorptions at 3427 cm-1& 3014 cm-1 suggest the presence of N-H group in [6] (Table-5). A strong band at 944 cm-1 is attributed to terminal Mo=O64, 67, 68stretching. NH2 bending absorption in the range 1159 cm−1-1182 cm−1 is also shifted to lower wave number 1108 cm−1, mainly due to coordination with molybdenum. Occurrence of terminal Mo=O is due to oxo abstraction59, 60 by molybdenum from THF.
Table 5: FTIR absorptions in cm−1
Absorptions |
1, 3-Diaminopropane74 |
[6] |
N-H str. |
3052–3351 |
3427.1 m, 3014.1 v s |
CH2 str. |
2873–2961 |
2898.3 sh, 2787.7 sh, 2701.1 sh |
NH2 bending |
1627 |
1609.1 s |
CH2 deformation |
1571–1582 |
1481.1 m, 1464.5 s, 1409.1 |
NH2 Bending |
1159–1182 |
1193.2 m, 1108.2 m |
C-N sym str. |
1062 |
1031.3 sh |
CH2 deformation |
742, 841 |
887.1 s, 786.1 m |
Mo-N |
449.3 w |
|
Terminal Mo=O64, 67, 68 str. |
944.9 s |
N-H stretching of pyrazole75, 76occursat 3452 cm-1. Band at 3386 cm-1 reflects that [7] contains N-H group. N-H stretching is declined due to N→Mo coordination. Medium band at 969 cm-1 shows the existence of terminal Mo=O64, 67, 68in [7] (Table-6). Occurrence of terminal Mo=O is due to oxo abstraction59, 60 by molybdenum from THF.
Table 6: FTIR absorptions in cm−1
Absorptions |
Pyrazole75, 76 |
[7] |
N-H, str. |
3452 |
3386.9 s |
C-H str. |
3153, 3142 |
3149.9 sh |
C=C ring str. |
1560 |
1631.0 m, 1519.0 sh |
N-C ring str. |
1467, 1140 |
1474.5 w, 1402.2 sh, 1350.3 w |
C-H in plane bending |
1047, 1037 |
1126.0 w, 1052.2 w |
C-H out of plane bending (wagging), ring twisting |
891, 841, 762 |
777.0 m, 760.1 sh, 740.8 sh |
Ring twisting |
658, 620 |
675.4 sh, 605.2 sh, 581.8 sh |
Mo-N |
437.7 sh |
|
Ring twisting, N-H wagging |
501 |
518.7 w |
Terminal Mo=O64, 67, 68 str. |
969.6 m |
1-Methylpyrrolidine77-79shows strong C-H symmetric stretching at2971cm-1 and C-H asymmetric stretching at2890 cm-1, 2832 cm-1, 2780 cm-1. C-H asymmetric stretching of [8] is found at 2750 cm-1. Weak band corresponding to the presence of terminal Mo=O64, 67, 68 str. is observed at 976 cm-1 in [8] (Table-7). A peak at 3410 cm-1 may be due to 1-methylpyrrolidinium cation. Occurrence of terminal Mo=O is due to oxo abstraction61, 62by molybdenum from THF.
Table 7: FTIR absorptions in cm−1
Absorptions |
1-Methylpyrrolodine77-79 |
[8] |
N+-H |
|
3410.6 v s, |
υs(C-H) str |
2971 s |
|
υas(C-H) str |
2890 sh, 2832 m, 2780 s |
2750.3 sh |
(C-H) deformation |
1450 s |
1634.0 m, 1462.0 w |
υ(C-C) str |
1364 s |
1383.7 sh |
υ(C-N) str |
1245 s, 1202 m, 1163 s, 1113 m |
1108.6 w |
(C-H) bending |
1046 s |
1004.6 sh |
CH2 rocking |
877 s |
734.9 m b |
CNC deformation |
575 w |
|
Terminal Mo=O64, 67, 68 str. |
976.1 w |
1H NMR Spectra
Spectra were taken in DMSO-d6solvent. Solvent residual peak ofDMSO-d680occurs at 2.50 ppm.THF80 spectrum in DMSO-d6shows O-CH2 peak and CH2peak at 3.60 ppm and 1.76 ppm, respectively. In the spectra given below, ↑ and ↓ represent upfield/downfield shift.
Succinimide51, 53 CH2 absorb at 2.73 ppm. Spectrum of [1]in DMSO-d6 shows CH2 absorption at 3.63 ppm showing downfield shift due to decrease in electron density around these protons on coordination with molybdenum through carbonyl group (Fig.-1, Table-8).
![]() |
Figure 1: 1H-NMR of Mo2O2Cl2(C4H5NO2)2(C4H8O)2, [1]. |
Spectrum of [2]in DMSO-d6 shows CH2 absorption at 3.42 ppm showing downfield shift due to decrease in electron density around these protons on coordination with molybdenum through carbonyl group (Fig.-2, Table-8).
![]() |
Figure 2: 1H-NMR of Mo2O3Cl5(C4H5NO2)2(C4H8O)2, [2]. |
Table 8: 1H NMR absorptions in ppm
Assignments |
Succinimide51, 53 in CDCl3 |
[1] |
[2] |
N-H |
8.9 |
11.06 ↓ |
10.97 ↓ |
CH2 |
2.73 |
3.63 ↓ |
3.42 ↓ |
Residual80 DMSO-d6 |
|
2.54 |
2.48 |
1, 4-Diaminobutane81in H2Oshows N-H peak at 1.15 ppm.NMR of [3] in DMSO-d6suggests that NH2 peak has shifted downfield. Peak of side CH2 (attached to N which coordinates)as well as peak of middle CH2 (attached to outer CH2 on the side in which N coordinates)have shifted downfield due to decrease in electron density around these protons on N→Mo coordination (Fig.-3, Table-9).
![]() |
Figure 3: 1H-NMR of Mo2O2Cl2(H2NCH2CH2CH2CH2NH2)2,[3]. |
Table 9: 1H NMR absorptions in ppm
Assignments |
1, 4-Diaminobutane81in H2O |
[3] |
NH2 |
1.14 4H |
8.23 ↓ |
Side CH2 (attached to N which coordinates) |
3.02-3.05 4H |
3.52 ↓ |
Middle CH2 (attached to outer CH2 on the side in which N coordinates) |
1.75-1.78 4H |
2.66 ↓ |
Residual80 DMSO-d6 |
|
2.43 |
Comparisonof 3-methylpyridine71, 72, 82spectrum withthat of [4], shows thatthere is downfield shift for all protons. This is due to reduction in ring π-electron density around these protons on sharing of lone pair by nitrogen with molybdenum (Fig.-4, Table-10).
![]() |
Figure 4: 1H-NMR of Mo3Cl8(C6H7N)4(C4H8O)2,[4]. |
Further, in the spectrum of [5], it is found that there is downfield shift for all protons. This is due to reduction in ring π-electron density around these protons on sharing of lone pair by nitrogen with molybdenum (Fig.-5, Table-10).
![]() |
Figure 5: 1H-NMR of Mo3O6Cl6(C6H7N)6,[5]. |
Table 10: 1H NMR absorptions in ppm
Absorptions |
3-Methylpyridine71, 72, 82 in CDCl3 |
[4] |
[5] |
H (CH3) |
2.32 3H Singlet |
3.27 ↓ |
2.56 ↓ |
H-C1 |
8.44 1H Singlet |
8.77 ↓ |
8.80 ↓ |
H-C3 |
7.45 1H Doublet |
8.40 ↓ |
8.23 ↓ |
H-C4 |
7.16 1H Triplet |
7.92 ↓ |
7.82 ↓ |
H-C5 |
8.42 1H Doublet |
8.70 ↓ |
8.74 ↓ |
Residual80 DMSO-d6 |
|
2.46 |
2.49 |
THF80 C-2, 5 (attached to N) |
|
3.53 |
|
THF80 C-3, 4 |
|
1.69 |
|
Comparison of spectrum of 1, 3-diaminopropane83 with that of [6] (Fig.-6, Table-11) in DMSO-d6suggests that NH2 and CH2 absorptions of 1, 3-diaminopropane have downfield shift. This is because of decrease in electron density around these protons on N→Mo coordination.
![]() |
Figure 6: 1H-NMR of MoO2Cl3(HNCH2CH2CH2NH2)2,[6] |
Table 11: 1H NMR absorptions in ppm
Assignments |
1, 3-Diaminopropane83in CDCl3 |
[6] |
NH2 |
1.21 4H |
7.89 ↓ |
Middle CH2 |
1.59 2H |
2.87 ↓ |
Side CH2 (attached to N which coordinates) |
2.76 4H |
3.56 ↓ |
Residual80 DMSO-d6 |
|
2.50 |
Spectrum of pyrazole72, 84 in CCl4 shows absorptions due to middle C-H proton at 6.31 ppm, C-H protons on other two carbons at 7.61 ppm and due to N-H proton at 12.64 ppm. Spectrum of [7] shows that all the pyrazole CH protons have moved downfield (Fig.-7, Table-12). This is because of decrease in electron density around these protons on N→Mo coordination. Due to keto-enol tautomerization equilibrium, peaks of CH protons of pyrazole appear as singlets.
![]() |
Figure 7: 1H-NMR of Mo2O4Cl4(C3H4N2)4,[7] |
Table 12: 1H NMR absorptions in ppm
Assignments |
Pyrazole72, 84in CCl4 |
[7] |
N-H |
12.64 1H |
9.42 ↑ |
Middle CH |
6.31 (s) 1H |
6.51 ↓ |
Side CH |
7.61(s) 2H |
7.96 ↓ |
Residual80 DMSO-d6 |
|
2.57 |
Spectrum of[8] shows that all the CH absorptions of 1-methylpyrrolidine77 have moved downfield, referring to decline in electron density of the ring on N→Mo coordination (Fig.-8, Table-13). There is a broad peak at 11.10 ppm indicating formation of 1-methylpyrrolidinium ion.
![]() |
Figure 8: 1H-NMR of Mo2O6Cl8(C5H11N)4, [8] |
Table 13: 1H NMR absorptions in ppm
Assignments |
1-Methylpyrrolidine77 |
[8] |
N+-H |
|
11.10 |
CH3 |
2.3 3H |
3.43-3.51 ↓ |
C2-H & C5-H (attached to N) |
2.5 4H |
2.73-2.91↓ |
C3-H & C4-H |
1.6 4H |
1.85-1.98 ↓ |
Residual80 DMSO-d6 |
|
2.50 |
Spectra were taken in DMSO-d6solvent. Solvent residual peak ofDMSO-d680occurs at 39.52±0.06 ppm.THF87 spectrum in DMSO-d6shows O-CH2 peak and CH2peak at 67.03 ppm and 25.14 ppm, respectively. In the spectra given below, ↑ and ↓ represent upfield/downfield shift.
Spectrum of [3] shows that there is slight upfield shift of all absorptions of 1, 4-diaminobutane85. This may be due to change of solvent from CDCl3 to DMSO-d6 (Fig.-9, Table-14).
![]() |
Figure 9: 13C-NMR of Mo2O2Cl2(H2NCH2CH2CH2CH2NH2)2,[3] |
Table 14: 13C NMR absorptions in ppm
Assignments |
1, 4-Diaminobutane85 in CDCl3 |
[3] |
C attached to nitrogen |
41 |
38.24↑ |
Other C |
29 |
25.04↑ |
Residual80 DMSO-d6 |
|
39.50 |
Spectrum of [4] shows that there is slight upfield shift of C-2 and C-6 of 3-methylpyridine86, whereas there is slight downward shift of C-3, C-4 and C-5. This is due to flow of π-electron density from C-3, C-4 and C-5 to N through C-2 and C-6, when N coordinates with Mo (Fig.-10, Table-15).
![]() |
Figure 10: 13C-NMR of Mo3Cl8(C6H7N)4(C4H8O)2,[4] |
Table 15: 13C NMR absorptions in ppm
Assignments |
3-Methylpyridine86 in CDCl3 |
[4] |
C-2 (attached to CH3) |
150.27 |
146.14 ↑ |
C-3 |
133.08 |
136.27 ↓ |
C-4 |
136.40 |
138.77 ↓ |
C-5 |
123.16 |
126.43 ↓ |
C-6 |
146.93 |
140.83 ↑ |
CH3 |
18.36 |
17.94 ↑ |
Residual80 DMSO-d6 |
|
39.42 |
THF87 C-2, 5 (attached to N) |
|
65.88 |
THF87 C-3, 4 |
|
23.97 |
Spectrum of [7] shows that there is practically no change in chemical shift of pyrazole88 on N→Mo coordination (Fig.-11, Table-16).
![]() |
Figure 11: 13C-NMR of Mo2O4Cl4(C3H4N2)4,[7] |
Table 16: 13C NMR absorptions in ppm
Assignments |
Pyrazole88 in CDCl3 |
[7] |
Carbons attached to N atoms |
134.0 |
133.12 ↑ |
Remaining carbon |
105.1 |
104.81 ↑ |
Residual80 DMSO-d6 |
|
39.42 |
Spectrum of [8] shows that there is slight upfield shift of all absorptions of 1-methylpyrrolidine89. This may be due to change of solvent from CDCl3 to DMSO-d6 (Fig.-12, Table-17).
![]() |
Figure 12: 13C-NMR of Mo2O6Cl8(C5H11N)4, [8] |
Table 17: 13C NMR absorptions in ppm
Assignments |
1-Methylpyrrolidine89 in CDCl3 |
[8] |
C-1, C-4 (attached to N) |
58.8 |
54.24 ↑ |
C-2, C-3 |
23.3 |
22.66 ↑ |
CH3 |
46.6 |
40.12 ↑ |
Residual80 DMSO-d6 |
|
39.42 |
Microbiological Activity
Molybdenum compounds prepared were tested using agar well diffusion assay methodfor their antibacterial and antifungal potential on the strains: Staphylococcus aureus (gram positive bacteria) (MTCC-737), E. coli (gram negative bacteria) (MTCC-1687), Candida albicans (fungus) (MTCC-227) and Aspergillus niger (fungus) (MTCC-282).Standard drugs amoxicillin and ketoconazole were used for bacteria and virus, respectively as reference.Zone of inhibition90 for a strain of bacteria/fungi was estimated to ascertain the amount of resistance of bacteria/fungi to the drug used as reference.Molybdenum compounds synthesized have been noted as potentially active against the above said bacteria and fungi (Table-18). Especially,
Compounds 1, 2, 4, 5 and 8 have greater antibacterial activity against E. coli than the reference drug (amoxicillin).
Compounds 1, 2 and 5 have greater antifungal activity against C. albicans than the reference drug (ketoconazole).
Table 18: Microbiological Study
Compound (100 µg/ml) |
Zone of inhibition90 (mm) |
|||
Gram- positive |
Gram- negative |
Antifungal |
||
S. aureus |
E. coli |
C. albicans |
A. niger |
|
Reference Drug |
25.69 |
18.35 |
21.37 |
28.21 |
[1] |
24.12 |
19.56 |
21.74 |
21.56 |
[2] |
19.28 |
22.51 |
23.12 |
21.58 |
[4] |
19.84 |
22.21 |
19.52 |
21.69 |
[5] |
21.54 |
49.62 |
21.47 |
19.87 |
[8] |
23.11 |
22.61 |
19.85 |
18.72 |
Conclusion and results: These compounds can kill and inhibit the growth of microbes |
Mass Spectra (LC-MS)
Theoretical m/z values of the fragments have been calculated91 on the basis of the most abundant isotopes of the individual elements. Fragments detected (Tables-19, 20) reinforce the formulae,
![]() |
Table 19: LC-MS Ionization. |
Table 20: LC-MS Ion m/z values
Comp. |
Fragment |
Calculated91 |
Detected |
Relative intensity |
[1] |
[MoOCl2(C4H8O)2]+ |
327.95 |
324.17 |
30% |
[MoOCl2(C4H5NO2)2(C4H8O)]2+ |
226.97 |
230.08 |
58% |
|
[MoOCl3(C4H5NO2)2(C4H8O)]+ |
488.92 |
490.35 |
8% |
|
[MoO2Cl2]+ |
199.83 |
197.04 |
34 |
|
[MoOCl2]2+ |
91.91 |
91.03 |
25 |
|
[Mo2O2Cl5(C4H5NO2)2(C4H8O)2]2+ |
372.41 |
376.20 |
100% |
|
[MoO2Cl2(C4H5NO2)(C4H8O)2]+ |
442.99 |
448.25 |
10% |
|
[2] |
[MoOCl2]2+ |
91.91 |
91.04 |
100 |
[C4H5NO2]+ |
99.03 |
100.05 |
21% |
|
[C4H8O]+ |
72.05 |
73.07 |
11% |
|
[MoOCl2(C4H5NO2)2(C4H8O)]2+ |
226.97 |
230.09 |
8% |
|
[MoOCl2(C4H5NO2)2(C4H8O)2]2+ |
263.00 |
263.13 |
52% |
|
[Mo2O2Cl2(C4H5NO2)2(C4H8O)2]2+ |
319.95 |
321.11 |
20% |
|
[MoO2Cl2(C4H5NO2)2(C4H8O)]+ |
469.95 |
471.24 |
12% |
|
[MoO2Cl2(C4H5NO2)2]+ |
397.89 |
397.23 |
18% |
|
[MoO2Cl2(C4H5NO2)]+ |
298.86 |
299.18 |
14% |
|
[MoO2Cl]+ |
164.86 |
163.10 |
15% |
|
[3] |
[MoO2Cl2]+ |
199.83 |
199.8 |
100% |
[MoO2Cl]+ |
164.86 |
164.90 |
40% |
|
[MoOCl]+ |
148.86 |
147.00 |
92% |
|
[MoO2]+ |
129.89 |
130.00 |
10% |
|
[H2NCH2CH2CH2CH2]+ |
72.08 |
71.0 |
22% |
|
[H2NCH2CH2CH2]+ |
58.06 |
59.0 |
36% |
|
[4] |
[C6H7N]+ |
93.05 |
94.07 |
44% |
[MoCl4]+ |
237.78 |
235.17 |
17% |
|
[MoCl4(C6H7N)]+ |
330.83 |
329.11 |
5% |
|
[Mo3Cl8(C6H7N)4]2+ |
472.84 |
472 |
1% |
|
[MoCl6(C6H7N)]+ |
400.77 |
400 |
3% |
|
[Mo3Cl8(C6H7N)4(C4H8O)2]2+ |
544.90 |
544.46 |
5% |
|
[Mo3Cl8(C6H7N)4(C4H8O)]2+ |
508.87 |
509.34 |
5% |
|
[C4H8O]+_ |
72.05 |
73.07 |
20% |
|
[5] |
[C6H7N]+ |
93.05 |
94.06 |
100 |
[MoO2Cl3(C6H7N)]+ |
327.85 |
328.25 |
10% |
|
[MoO2Cl3(C6H7N)]2+ |
163.92 |
163.0 |
1% |
|
[MoO2Cl3(C6H7N)3]2+ |
256.98 |
256.18 |
5% |
|
[Mo3O2Cl3(C6H7N)4]2+ |
401.40 |
400.31 |
5% |
|
[Mo3O5Cl3(C6H7N)5]2+ |
471.94 |
472.38 |
3% |
|
[MoOCl2]2+ |
91.91 |
91.04 |
3% |
|
[Mo3O6Cl4(C6H7N)6]2+ |
543.95 |
545.49 |
2% |
|
[Mo3O6Cl6(C6H7N)6]2+ |
578.92 |
581.38 |
<1% |
|
[6] |
[MoO(HNCH2CH2CH2NH2)]+ |
186.97 |
187.00 |
15% |
[MoO(HNCH2CH2CH2NH2)]2+ |
93.48 |
93.10 |
60% |
|
[MoOCl(HNCH2CH2CH2NH2)]+ |
221.94 |
220.0 |
100.0% |
|
[H2NCH2CH2CH2]+ |
58.06 |
59.0 |
4% |
|
[7] |
[C3H4N2]+ |
68.03 |
69.05 |
100% |
[MoOCl2]2+ |
91.91 |
91.04 |
27% |
|
[MoO2Cl]+ |
164.86 |
163.11 |
24% |
|
[MoO2Cl3(C3H4N2)2]+ |
370.87 |
375.30 |
5% |
|
[MoO2Cl3]+ |
234.80 |
235.17 |
8% |
|
[Mo2O3Cl4(C3H4N2)2]+ |
519.74 |
519.42 |
3% |
|
[Mo2O3Cl2(C3H4N2)2]+ |
449.80 |
447.36 |
5% |
|
[8] |
[C5H11N]+ |
85.08 |
86.09 |
100% |
[MoOCl2]2+ |
91.91 |
91.04 |
3% |
|
[Mo2O3(C5H11N)2]2+ |
206.98 |
207.19 |
6% |
|
[Mo2O6Cl4(C5H11N)2]+ |
601.83 |
596.19 |
2% |
|
[MoO2Cl]+ |
164.86 |
163.12 |
2% |
|
[Mo2O5Cl4(C5H11N)]+ |
500.74 |
503.10 |
7% |
Conclusion
Band at 3294 cm-1 indicates that [1] contains succinimide N-H group. Bands at 980 cm-1and 919 cm-1support the availability of cis-MoO22+core in [1]. Occurrence of cis-MoO22+ core is due to oxo abstraction by molybdenum from THF. There is decrease in C=O sym and asym absorptions due to decrease in C=O bond order on O→Mo coordination in [1]. Succinimide CH2 absorb at 2.73 ppm. Spectrum of [1]shows CH2 absorption at 3.63 ppm showing downfield shift due to decrease in electron density around these protons on coordination with molybdenum through carbonyl group. Microbiological studies reveal that [1] is effective against the bacteria/fungi tested for, especially E. coli and C. albicans, where [1] is more effective than the reference drugs themselves. Elemental analysis and LC-MS fragmentation support the proposed formula.
Band at 3433 cm-1 indicates that [2] contains succinimide N-H group. Bands at 984 cm-1and 923 cm-1support the availability of cis-MoO22+ core in [2]. Occurrence of cis-MoO22+ core is due to oxo abstraction by molybdenum from THF. There is decrease in C=O sym and asym absorptions due to decrease in C=O bond orderon O→Mo coordination in [2]. Spectrum of [2]shows CH2 absorption at 3.42 ppm showing downfield shift due to decrease in electron density around these protons on coordination with molybdenum through carbonyl group. Microbiological studies reveal that [2] is effective against the bacteria/fungi tested for, especially E. coli and C. albicans, where [2] is more effective than the reference drugs themselves. Elemental analysis and LC-MS fragmentation support the proposed formula.
Strong 1, 4-diaminobutaneN-H absorptions have been recorded at 3391 cm-1, 3077 cm-1 and 3010 cm-1 in [3]. Terminal Mo=O stretching occurs at 990 cm-1 -1010 cm-1 in various inert solvents. A medium Mo=O stretching at 921 cm-1 conforms to the presence of terminal Mo=O group. There is a decline in Mo=O stretching to 921 cm-1 showing Mo coordination to 1, 4-diaminobutane through N atom, in a direction trans to Mo=O bond. Bending mode due to NH2 observed in 1, 4-diaminobutane at 1146 cm−1 is declined to 1116 cm−1, because of N→Mo coordination. 1, 4-Diaminobutaneshows N-H peak at 1.15 ppm.NMR of [3] suggests that NH2 peak has shifted downfield. Peak of side CH2 (attached to N which coordinates)as well as peak of middle CH2 (attached to outer CH2 on the side in which N coordinates)have shifted downfield due to decrease in electron density around these protons on N→Mo coordination. 13CNMR spectrum of [3] shows that there is slight upfield shift of all absorptions of 1, 4-diaminobutane . This may be due to change of solvent from CDCl3 to DMSO-d6. Elemental analysis and LC-MS fragmentation support the proposed formula.
Strong bands at 3119 cm-1 and 3054 cm-1 have been noticed in [4] which show presence of 3-methylpyridinering C-H absorptions. Ring C=N stretching & ring C=N torsion wave numbers have increased and ring C-H bending mode wave numbers have declined due to Mo(dπ)→N(pπ) back bonding. A strong band at 989 cm-1 revealsthe presence of terminal Mo=O in [4].Comparisonof 3-methylpyridinespectrum withthat of [4], shows thatthere is downfield shift for all protons. This is due to reduction in ring π-electron density around these protons on sharing of lone pair by nitrogen with molybdenum.13C NMR spectrum of [4] shows that there is slight upfield shift of C-2 and C-6 of 3-methylpyridine, whereas there is slight downward shift of C-3, C-4 and C-5. This is due to flow of π-electron density from C-3, C-4 and C-5 to N, through C-2 and C-6, when N coordinates with Mo. Microbiological studies reveal that [4] is effective against the bacteria/fungi tested for, especially E. coli where [4] is more effective than the reference drug itself. Elemental analysis and LC-MS fragmentation support the proposed formula.
Strong band at 3391 cm-1 has been noticed in [5] which shows presence of 3-methylpyridinering C-H absorption. Ring C=N stretching & ring C=N torsion wave numbers have increased and ring C-H bending mode wave numbers have declined due to Mo(dπ)→N(pπ) back bonding. A medium band at 949 cm-1 reveals the presence of terminal Mo=O group in [5].Comparison of 3-methylpyridine spectrum with that of [5], shows thatthere is downfield shift for all protons. This is due to reduction in ring π-electron density around these protons on sharing of lone pair by nitrogen with molybdenum. Microbiological studies reveal that [5] is effective against the bacteria/fungi tested for, especially E. coli and C. albicans, where [5] is more effective than the reference drugs themselves. Elemental analysis and LC-MS fragmentation support the proposed formula.
Absorptions at 3427 cm-1& 3014 cm-1 in [6] suggest the presence of 1, 3-diaminopropane N-H group in the compound. A strong band at 944 cm-1 is attributed to terminal Mo=O stretching. NH2 bending absorption in the range of 1159 cm−1-1182 cm−1 is also shifted to lower wave number 1108 cm−1, mainly due to coordination with molybdenum. Occurrence of terminal Mo=O is due to oxo abstraction by molybdenum from THF. Comparison of spectrum of 1, 3-diaminopropane with that of [6]suggests that NH2 and CH2 absorptions of 1, 3-diaminopropane have downfield shift. This is because of decrease in electron density around these protons on N→Mo coordination. Elemental analysis and LC-MS fragmentation support the proposed formula.
Band at 3386 cm-1 reflects that [7] contains pyrazole N-H group. N-H stretching is declined due to Mo-N coordination. Medium band at 969 cm-1 shows the existence of terminal Mo=O in [7]. Occurrence of terminal Mo=O is due to oxo abstraction by molybdenum from THF. Spectrum of pyrazole shows absorptions due to middle C-H proton at 6.31 ppm, C-H protons on other two carbons at 7.61 ppm and due to N-H proton at 12.64 ppm. Spectrum of [7] shows that all the pyrazole CH protons have moved downfield. This is because of decrease in electron density around these protons on N→Mo coordination. Due to keto-enol tautomerization equilibrium, peaks of CH protons of pyrazole appear as singlets.13 C NMR spectrum of [7] shows that there is practically no change in chemical shift of pyrazole. Elemental analysis and LC-MS fragmentation support the proposed formula.
Absorption at 2750 cm-1 in [8] shows the presence of 1-methylpyrrolidine C-H asymmetric stretching. Weak band corresponding to the presence of terminal Mo=O is observed at 976 cm-1. A peak at 3410 cm-1 may be due to 1-methylpyrrolidinium cation. Occurrence of terminal Mo=O is due to oxo abstraction by molybdenum from THF. Spectrum of[8] shows that all the CH absorptions of 1-methylpyrrolidinehave moved downfield, referring to decline in electron density of the ring on N→Mo coordination. There is a broad peak at 11.10 ppm indicating formation of 1-methylpyrrolidinium ion.13C NMR spectrum of [8] shows that there is slight upfield shift of all absorptions of 1-methylpyrrolidine. This may be due to change of solvent from CDCl3 to DMSO-d6. Microbiological studies reveal that [8] is effective against the bacteria/fungi tested for, especially E. coli where [8] is more effective than the reference drug itself. Elemental analysis and LC-MS fragmentation support the proposed formula.
Acknowledgement
We, the authors thank P. U. Chandigarh, India for providing facility of elemental analysis and spectral studies. We are also thankful to ISF Analytical Laboratory (ISF College of Pharmacy), Ferozepur Road, Moga, Punjab (India) for carrying out microbiological studies.
Conflict of Interest
There is no conflict of interest among the authors.
References
- Shetgiri, N. P.; Nayak,B. K., Indian J. Chem., 2005, 44B, 1933-1936.
- Aeberli, P; Go gerty, J. H; Houlihan, W. J; Iorio, L. C., J. Med. Chem.,1976, 19(3), 436-438.
CrossRef - Pennington, F. C.; Guercio, P. A.; Solomons, I. A., J. Amer. Chem. Soc., 1953, 75(9), 2261.
CrossRef - Hall, I. H.; Wong, O. T.; Scovill, J. P., Biomed Pharmacother, 1995, 49(5), 251-258.
CrossRef - Crider, A. M; Kolczynski, T. M.; Yates, K. M., J. Med. Chem.,1980, 23(3), 324-326.
CrossRef - Johnston, T. P.; Piper, J. R.; Stringfellow, C. R., J. Med. Chem., 1971, 14(4), 350-354.
CrossRef - Kaczorowski, G. J.; McManus, O. B.; Priest, B. T.; Garcia, M. L., Gen. Physiology, 2008, 131(5), 399-405.
CrossRef - Musso, D. L.; Cochran, F. R.; Kelley, J. L.; McLean, E. W.; Selph, J. L.; Rigdon, G. C., J. Med. Chem., 2003, 46(3), 399-408.
CrossRef - Rich, D. H.; Gardner, J. H., Tetrahedron Letters, 1983, 24(48), 5305-5308.
CrossRef - Zentz, F.; Valla, A.; Guillou, R. L.; Labia, R.; Mathot, A. G.; Sirot, D., Farmaco, 2002, 57(5), 421-426.
CrossRef - Correa, R.; Filho, V. C.; Rosa, P. W.; Pereira, C. I.; Schlemper, V.; Nunes, R. J., Pharm. Pharmacol. Comm., 1997, 3(2), 67-71.
- Kornet, M. J.; Crider, A. M.; Magarian, E. O., J. Med. Chem., 1977, 20(3), 405-409.
CrossRef - Isaka, M.; Prathumpai, W.; Wongsa, P.; Tanticharoen, M.; Hirsutellone, F., Org. Lett.,2006, 8(13), 2815-2817.
CrossRef - Filho, V. C.; Nunes, R. J.; Calixto, J. B.; Yunes, R. A., Pharm. Pharmacol. Comm., 1995, 1(8), 399-401.
- Hazra, B. G.; Pore, V. S.; Day, S. K.; Datta, S.; Darokar, M. P., Saikia, D., Bioorg. Med. Chem. Lett., 2004, 14(3), 773-777.
CrossRef - Amah, K. U.; Sylvain, A. Y. G.; Gaston, K. A.; Alice, K. H. M. T.; Baptiste,M. J.,International Research Journal of Pure & Applied Chemistry, 2016, 12(4), 1-11.
CrossRef - Gao, S.; Wang, J.; Xu, S.; Li, H.; Chen, K.; Ouyang, P., Chinese Journal of Chemical Engineering, 2021, 30, 4-13.
CrossRef - Gaymans, R. J.; Utteren, T. E. C.; van den Berg, J. W. A.; Schuyer, J., Journal of Polymer Science: Polymer Chemistry Edition, 1977, 15(3), 537-545.
CrossRef - Pasupathy, A.; Nirmala, S.; Abirami, G.; Satish, A.; Milton, R. P., International Journal of Scientific and Research Publications, 2014, 4(3), 1-3.
- Stellman, J. M., Encyclopaedia of Occupational Health and Safety, 4th Edition, International Labour Office, Geneva, 1998, 4.
- Shimizu, S.; Watanabe, N.; Kataoka, T.; Shoji, T.; Abe, N.; Morishita, S.; Ichimura. H., Ullmann’s Encyclopedia of Industrial Chemistry, 2002, doi:10.1002/14356007.a22_399.
CrossRef - Sims, G. K.; Sommers, L. E., Environmental Toxicology and Chemistry, 1986, 5, 503-509.
CrossRef - Sims, G. K.; Sommers, L. E., J. Environmental Quality, 1985, 14, 580-584.
CrossRef - Srinivasu, J. V.; Narendra, K.; Rao, B. S., Indian Journal of Pure & Applied Physics, 2017, 55, 797-805.
- Bingham, E.; Cohrssen, B., Patty’ Toxicology, John Wiley & Sons, 6th Edition, 2012, 1.
- Chae, T. U.; Kim, W. J.; Choi, S.; Park, S. J.; Lee, S. Y., Scientific Reports, 2015, 5, article 23040, 1-13.
CrossRef - Abebe, A.; Bayeh, Y.; Belay, M.; Gebretsadik, T.; Thomas, M.; Linert, W., Future Journal of Pharmaceutical Sciences, 2020, 6, Article No. 13, 1-9.
CrossRef - Salim, E. I.; Wanibuchi, H.; Morimura, K.; Kim, S.; Yano, Y.; Yamamoto, S.; Fukushima S., Carcinogenesis, 2000, 21(2), 195-203.
- J. E. Seely; Pegg, A. E., Biochem. J., 1983, 216, 701-707.
CrossRef - Barcelo, M.; Ravina, E.; Masaguer, C. F.; Dominguez, E.; Areias, F. M.; Brea, J., Bioorg. Med. Chem. Lett.,2007, 17, 4873-4877.
CrossRef - Pospisil, P.; Folkers, G.; FABAD J. Pharm. Sci.,2004, 29, 81-92.
- Cho, A. E.; Guallar, V.; Berne, B. J.; Friesner, R., J. Computchem.,2005, 26, 915-931.
CrossRef - Bekhit, A. A.; Ashour, H. M. A.; Ghang, Y. S. A.; Bekhit, A. E. A.; Baraka, A., Eur. J. Med. Chem., 2008,43, 456-463.
CrossRef - Aziz, M. A.; Abuorahma, G. E. A.; Hassan, A. A., Eur. J. Med. Chem.,2009, 44, 3480-3487.
CrossRef - Ahmed, O. M.; Muhamed, M. A.; Ahmed, R. R.; Ahmed, S. A., Eur. J. Med. Chem., 2009, 44, 3519-3523.
CrossRef - Frigola, J.; Colombo, A.; Pares, J.; Martinez, L.; Sagarra, R.; Rosert, R., Eur. J. Med. Chem., 1989,24, 435-445.
CrossRef - Bondock, S.; Rabie, R.; Etman, H. A.; Fadda, A. A., Eur. J. Med. Chem.,2008, 43, 2122-2229.
CrossRef - Castagnolo, D.; Mantti, F.; Radi, M.; Bechi, B.; Pagano, M.; Logu, A. D., Bioorg. Med. Chem., 2009,17, 5716-5721.
CrossRef - Gopalakrishnan, S.; Ravi, T. K.; Manojkumar, P., Eur. J. Med. Chem.,2009, 44, 4690-4694.
CrossRef - Bekhit, A. A.; Aziem, T. A., Bioorg. Med. Chem., 2004, 12, 1935-1945.
CrossRef - Richardson, C. H.; Shepard, H. H., Journal of Agricultural Research, 1930, 40(11), 1007-1015.
- Page, N.; Stevenson, R.; Powell, M., Analytical Methods, 2014, 6(4), 1248-1253.
CrossRef - Abebe, A.; Hailemariam, T., Bioinorganic Chemistry and Applications, 2016, Article ID 3607924, 1-9.
CrossRef - Thomas, D. D.; Ridnour, L. A.; Isenberg, J. S.; Flores, S. W.; Switzer, C. H.; Donzelli, S.; Hussain, P.; Vecoli, C.; Paolocci, N.; Ambs, S.; Colton, C. A.; Harris, C. C.; Roberts, D. D.; Wink, D. A., Free Radical Biology and Medicine, 2008, 45(1), 18-31.
CrossRef - Chen, P. R.; He, C., Current Opinion in Chemical Biology, 2008, 12(2), 214-21.
CrossRef - Pennella, M. A.; Giedroc, D. P., Biometals, 2005, 18(4), 413-28.
CrossRef - Cowan, J. A.; Bertini, I.; Gray, H. B.; Stiefel, E. I.; Valentine, J. S.,
Structure and Reactivity: Biological Inorganic Chemistry, 3, University Science Books, Sausalito, 2007, 8(2): 175181. - Jameel, A.; MSA, S. A. P., Asian Journal of Chemistry, 2010, 22(12), 3422-48.
- Anupama, B.; Sunuta, M.; Leela, D. S.; Ushaiah; Kumari, C. G., Journal of Fluorescence, 2014, 24(4), 1067-76.
CrossRef - Singh, G.; Mangla, V.; Goyal, M.; Singla, K.; Rani, D., American International Journal of Research in Science, Technology, Engineering & Mathematics, 2014, 8(2), 131-136.
- Singh, G.; Mangla, V.; Goyal, M.; Singla, K.; Rani, D., American International Journal of Research in Science, Technology, Engineering & Mathematics, 2015,10(4), 299-308.
- Singh, G.; Mangla, V.; Goyal, M.; Singla, K.; Rani, D., American International Journal of Research in Science, Technology, Engineering & Mathematics, 2015,11(2), 158-166.
- Singh, G.; Mangla, V.; Goyal, M.; Singla, K.; Rani, D.; Kumar, R., American International Journal of Research in Science, Technology, Engineering & Mathematics, 2016, 16(1), 56-64.
- Singh, G.; Kumar, R., American International Journal of Research in Science, Technology, Engineering & Mathematics, 2018, 22(1), 01-08.
- Rani, D.; Singh, G.; Sharma, S., Oriental Journal of Chemistry, 2020, 36(6), 1096-1102.
CrossRef - Singh, G.; Mangla, V.; Goyal, M.; Singla, K.; Rani, D., International Congress on Chemical, Biological and Environmental Sciences,2015, 930-942, May 7-9, Kyoto (Japan).
- Rani, D.; Singh, G.; Sharma, S., Oriental Journal of Chemistry, 2021, 37(1), 46-52.
CrossRef - Rani, D.; Singh, G.; Sharma, S., Oriental Journal of Chemistry, 2021, 37(2), 459-466.
CrossRef - Planinic, P.; Meider, H.; Yeh, H.; Vikic-Topic, D., J. Coord. Chem., 1992, 25, 193-204.
CrossRef - Behzadi, K.; Baghlaf, A. O.; Thompson, A., J. Less Common Metals, 1978, 57, 103-110.
CrossRef - Vogel, A. I., A Text Book of Quantitative Inorganic Analysis; John Wiley and Sons: New York, (Standard methods), 1963.
- Stamboliyska, B. A.; Binev, Y. I.; Radomirska, V. B.; Tsenov, J. A.; Juchnovskiet, I. N., Journal of Molecular Structure, 2000, 516, 237-245.
CrossRef - Uno, T.; Machida, K., Bulletin of the Chemical Society of Japan, 1962, 35(2),276-283.
CrossRef - Heyn, B.; Hoffmann; Regina, Z. Chem.,1976, 16, 407.
CrossRef - Abramenko, V. L.; Sergienko, V. S.; Churakov, A.V., Russian J. Coord. Chem., 2000, 26(12), 866-871.
CrossRef - Ergu¨ N. Kasap; Su¨ Leyman; O¨ Zceli´K., J. Inclusion Phenomena and Molecular Recognition in Chem., 1997, 28, 259-267.
CrossRef - Barraclough, C. G.; Kew, D. J., Australian J. Chem., 1970, 23, 2387-2396.
CrossRef - Ward, B. G.; Stafford, F. E., Inorg. Chem.,1968,7, 2569.
CrossRef - Abramenko, V. L.; Sergienko, V. S., Russian J. Inorg. Chem., 2009, 54(13), 2031-2053.
CrossRef - Toco’n, I. L.; Woolley, M. S.; Otero, J. C.; Marcos, J. I., Journal of Molecular Structure, 1998,470, 241-246.
CrossRef - Gupta, S. K.; Srivastava, T. S., J. Inorganic and Nuclear Chem., 1970, 32, 1611-1615.
CrossRef - Hossain, A. G. M. M.; Ogura, K., Indian J. Chem., 1996, 35A, 373-378.
- Brewerp, D. G.; Wong, P. T. T.; Sears, M. C., Canadian J. Chem., 1968, 46(20), 3119-3128.
CrossRef - Yadav, S.; Moheman, A.; Siddiqi, K. S., Arabian Journal of Chemistry, 2016, 9, suppliment 2, S1747-S1754.
CrossRef - Stamboliyska, B. A.; Binev, Y. I.; Radomirska, V. B.; Tsenov, J. A.; Juchnovski, I. N., Journal of Molecular Structure,2000,516, 237-245.
CrossRef - Uno, T.; Machida, K.,Bulletin of the Chemical Society of Japan, 1962, 35(2), 276-283.
CrossRef - Hoa, N. V.; Tuan, N. A.; Thao, P. T.; Huyen, T. T. T., Journal of Science and Technology, 2016, 54(2), 231-237.
- Wang, G. T.; Mui. C.; Tannci, J. F.; Filler, M. A.; Musgrave, C. B.; Bent, S. F., J. Phys. Chem., B, 2003, 107, 4982-4996.
CrossRef - Szafran, M.; Koput, J.; Szafran, Z. D.; Kwiatkowski, J. S., Vibrational Spectroscopy., 2000, 23, 1-11.
CrossRef - Gottlieb, H. E.: Kotlyar, V.; Nudelman, A., J. Org. Chem., 1997, 62, 7512-7515.
CrossRef - Olmo, C.; Casas, M. T.; Martínez, J. C.; Franco, Puiggalí, L.; J., Polymers, 2019, 11(4), 572, 1-19.
CrossRef - Kumari, N.; Sharma, M.; Das,, P.; Dutta, D. K., Applied Organomet. Chem., 2002, 16, 258-264.
CrossRef - Chatterjee, C.; Phulambrikar, A.; Das, S., J. Coord. Chem., 1990, 21(3), 231-236.
CrossRef - Editor: Teresa M. V. D. Pinho e Melo, Recent Research Developments in Heterocyclic Chemistry,: 397-475 ISBN: 81-308-0169-8, 2007.
- https://spectrabase.com/compound/GhReRxNt2Lp#9R7ID3SyH4X.
- https://www.chemicalbook.com/SpectrumEN_108-99-6_13CNMR.htm.
- Babij, Ni. R.; E. O.; McCusker, Whiteker, G. T.;Canturk, B.; Choy, N.; Creemer, L. C.; Amicis, C. V. D.; Hewlett, N. M.; Johnson, P. L.; Knobelsdorf, J. A.; Li, F.; Lorsbach, B. A.; Nugent, B. M.; Ryan, S. J.; Smith, M. R.; Yang, Q., Org. Process Res. Dev., 2016, 20, 661-667.
CrossRef - Begtrup, M.; Boyer, G.; Cabildo, P.; Cativiela, C.; Claramunt, R. M.; Elguero, J.; Garcia, J. I.; Toiron, C.; Vedser, P., Magnetic Resonance in Chemistry, 1993, 31, 107-168.
CrossRef - http://www.molbase.com/en/hnmr_120-94-5-moldata-22594.html.
CrossRef - Bhattacharjee, M. K., The Journal of Antibiotics, 2015, 68, 657-659.
CrossRef - Audi, G.; Wapstra, A. H., Nucl. Phys. A. 1995, 595, 409-480.
CrossRef
This work is licensed under a Creative Commons Attribution 4.0 International License.
0 Comments