Reactions of MoOCl4 and MoO2Cl2 with Heterocyclic Thioamides
Deepika Rani1, Gursharan Singh2* and and Seema Sharma2
1Punjab Technical University, Kapurthala India.
2Department of Applied Chemistry, Giani Zail Singh Campus College of Engineering & Technology,Dabwali Road, MRSPTU Bathinda-151001-India.
Corresponding Author E-mail: gursharans82@gmail.com
DOI : http://dx.doi.org/10.13005/ojc/370228
Article Received on : 17-Mar-2021
Article Accepted on :
Article Published : 12 Apr 2021
MoOCl4/MoO2Cl2 were reacted with 2-mercaptopyridine (IUPAC: pyridine-2-thiol)/4-phenylimidazole-2-thiol (IUPAC: 4-phenyl-1, 3-dihydroimidazole-2-thione)/6-mercaptopurine (IUPAC: 1, 7-dihydro-purine-6-thione) in acetonitrile medium using equal/twice molar concentrations at normal temperature. The reactions yielded products: MoOCl3(C5H4NS-SNH4C5).2HCl, [1]; Mo3O3Cl12(C5H4NS-SNH4C5)(CH3CN)2, [2]; Mo2OCl6(C9H7N2S)4, [3] and Mo2O2Cl8(C5H4N4S)(CH3CN), [4].
The various techniques used for characterization of compounds are: Fourier transform infrared, proton nuclear magnetic resonance, 13C nuclear magnetic resonance, liquid/gas chromatography mass spectrometry and C, H, N, S, Mo, Cl analysis. The products are prone to oxidation/hydrolysis by air/moisture, so all procedures were executed in vacuum line using dry nitrogen atmosphere. Elemental analysis and fragments recorded in mass spectrometry are in tune with the formulae proposed.
Acetonitrile Medium; 13C NMR; DMSO-d6; FTIR; Liquid/Gas Chromatography-Mass Spectrometry; 2-mercaptopyridine (IUPAC: pyridine-2-thiol), 4-phenylimidazole-2-thiol (IUPAC: 4-phenyl-1, 3-dihydroimidazole-2-thione), 6-mercaptopurine (IUPAC: 1, 7-dihydro-purine-6-thione), MoOCl4, MoO2Cl2
Download this article as:
Copy the following to cite this article: Rani D, Singh G, Sharma S. Reactions of MoOCl4 and MoO2Cl2 with Heterocyclic Thioamides. Orient J Chem 2021;37(2). |
Copy the following to cite this URL: Rani D, Singh G, Sharma S. Reactions of MoOCl4 and MoO2Cl2 with Heterocyclic Thioamides. Orient J Chem 2021;37(2). Available from: https://bit.ly/3mG2eEC |
Introduction
6-Mercaptopurine (IUPAC: 1, 7-dihydro-purine-6-thione)
Pyrimidine ring and imidazole ring are fused in purine. 6-mercaptopurine (mpH) has delocalized π-electrons. Nucleophiles can attack at positions 2, 6 and 8. Electrophiles can attack at positions 3 and 7.
Purine ring system represents skeleton for nucleic acid bases guanine and adenine. 6-Mercaptopurine1-2 is used as chemotherapy drugs to treat various types of cancers. This drug is also used to cure autoimmune diseases. 6-Mercaptopurine is marketed as purinethol. Purine is antagonists drug because it stops the growth of cancer cells.
6-Mercaptopurine reacts with many transition metals3-4. Some transition metal complexes of the ligand are more potent against cancer than the ligand itself5-8. 6-Mercaptopurine coordinates5, 7, 9 via sulphur and nitrogen atoms with divalent transition metals. 6-Mercaptopurine (mpH) reacts with various transition metal ions in different coordination ways: mpH+, mp–, mp2- and intramolecular proton transfer. There is thione-thiol tautomerism. In gaseous state, thiol form is favoured, whereas in solution form thione form is favoured10. There is also prototropic tautomerism10 in 6-mercaptopurine between 7 and 9 positions.
![]() |
Scheme 1: 6-Mercaptopurine Click here to View scheme |
4-Phenylimidazole-2-thiol (IUPAC: 4-phenyl-1, 3-dihydroimidazole-2-thione)
Thioamides have higher reactivity11 than ordinary amides, because (a) thioamides have lesser C=S double bond character than C=O due to larger size of S atom than that of C atom and bond dissociation energy of C=O bond is 50 k.cal./mole higher than that of C=S bond, (b) greater participation of the sulphur to lower occupied molecular orbitals of the thioamide group, (c) there is more electron density on nitrogen atom in thioamides, so protons on the nitrogen atom are more labile, (d) higher lability of the protons on carbons next to the thioamide group. The sulphur atom of thioamide group accepts nucleophiles and electrophiles.
Heterocyclic thioamides are used in anti-thyroidal drugs12. Heterocyclic imidazothiazole are biologically active compounds13. They are able to inhibit many receptors and enzymes14-17. They have applications as diuretic18, antihelmintic19, fungicidal20, antidiabetic21,antitumor22-27 andantimicrobial28-29 drugs. Thioligand metal complexes have biochemical applications30-32. They are also used as analytical reagents or metal corrosion inhibitors12.
![]() |
Scheme 2: 4-Phenylimidazole-2-thiol Click here to View scheme |
2-Mercaptopyridine (IUPAC: pyridine-2-thiol)
2-Mercaptopyridine and 1H-pyridine-2-thione are tautomers. We can represent 2-mercaptopyridine as pySH. There are two probable coordination sites11 pySH and pyS– (pyridine-2-thiolate ion), but pySH is more prevalent with transition metals. pyS– can also act as bridging ligand. pyS– (pyridine-2-thiolate ion) further tautomerises11 to pyridine-2-thioamidate ion with negative charge on nitrogen atom.
![]() |
Scheme 3: 2-Mercaptopyridine Click here to View Scheme |
Aim of Investigation
Many ligands have been found to react with MoO2Cl2 and MoOCl4.
Many complexes of alkanediols, amides, aromatic azoles, diaminoalkanes, imides, purine, thioamides and thiols with MoO2Cl2 have been reported33-40 by the author.
Many complexes of alkylpyridines, amides, alkylpyrrolidine, alkylpiperidine, aromatic azoles, diaminoalkanes, imides, mercaptopyridine, mercaptopyridine-N-oxide sodium, purine and 2-thiazoline-2-thiol with MoOCl4 have also been reported33-35, 40-42 by the author.
Since complexes of these ligands with transition metals have many applications, we have synthesized complexes of 2-mercaptopyridine (IUPAC: pyridine-2-thiol)/4-phenylimidazole-2-thiol (IUPAC: 4-phenyl-1, 3-dihydroimidazole-2-thione)/6-mercaptopurine (IUPAC: 1, 7-dihydro-purine-6-thione) with precursors MoOCl4 and MoO2Cl2. The various techniques used for characterization of compounds are: Fourier transform infrared, proton nuclear magnetic resonance, 13C nuclear magnetic resonance, liquid/gas chromatography mass spectrometry and C, H, N, S, Mo, Cl analysis. The products are prone to oxidation/hydrolysis by air/moisture, so all procedures were executed in vacuum line using dry nitrogen atmosphere.
Materials and Methods
Synthesis of MoOCl4 was carried out from thionyl chloride and molybdenum trioxide at reflux temperature. Unreacted thionyl chloride was evacuated into liquid nitrogen cooled traps. Dark green crystals of MoOCl4 isolated on evacuation were dissolved in dry dichloromethane. Dark red solution was obtained. It was filtered to eliminate any unreacted/undissolved MoO3. Red solution was evaporated to get dark green crystals of MoOCl4.
2-Mercaptopyridine, 4-phenylimidazole-2-thiol, 6-mercaptopurine monohydrate and MoO2Cl2were procured from Sigma-Aldrich and used as such.
The products are prone to oxidation/hydrolysis by air/moisture, so all procedures were executed in vacuum line using dry nitrogen atmosphere. Ligand solution in dry acetonitrile was added drop wise to MoOCl4/MoO2Cl2 with continuous stirring from pressure stabilised dropping funnel. The reactants were agitated for 6-7 h. The products were filtered through G-4 sintered glass crucible filtration unit and isolated.
Oxinate43 gravimetric method was used in the lab for molybdenum estimation. Silver chloride43 gravimetric method was used in the lab for chlorine estimation. Remaining elements were analysed with Thermo Finnigan Elemental Analyser. Infrared spectra were recorded with Perkin-Elmer 400 FTIR Spectrometer. Proton nuclear magnetic resonance, 13C nuclear magnetic resonance spectra in DMSO-d6 were taken with Multinuclear Brucker Avance-II 400 NMR spectrometer. Liquid/Gas Chromatography-Mass spectra were recorded in the range 0 – 1100 m/z. Above instruments were used at P. U. Chandigarh.
Reactions
It is probable that mechanism of these reactions involve oxidation/reduction, disproportionation/rearrangement, isomerization steps. The product source residue (R) or filtrate (F) is shown below the products.
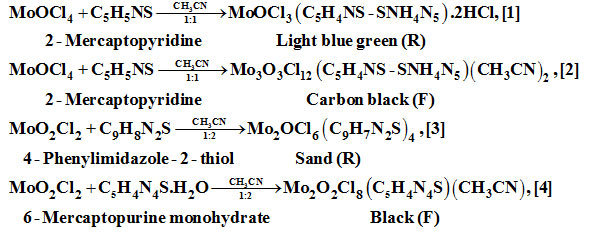
Results and Discussions
Analytical Measurements
Table 1: shows the percentage of the elements. Theoretical values are enclosed in brackets.
(Elemental Analysis) |
||||||
Compounds |
Cl |
Mo |
H |
C |
S |
N |
MoOCl3(C5H4NS-SNH4C5).2HCl, [1] (Light blue green/511.5) |
34.02 (34.70) |
17.93 (18.76) |
02.50 (01.95) |
22.78 (23.46) |
11.87 (12.51) |
05.11 (5.47) |
Mo3O3Cl12(C5H4NS-SNH4C5)(CH3CN)2, [2] (Carbon black/1064.0) |
39.78 (40.03) |
27.59 (27.06) |
01.95 (01.31) |
15.58 (15.78) |
05.67 (06.01) |
04.77 (5.26) |
Mo2OCl6(C9H7N2S)4, [3] (Sand/1121.0) |
19.78 (19.00) |
16.28 (17.12) |
02.88 (02.49) |
38.97 (38.53) |
12.32 (11.41) |
10.08 (9.99) |
Mo2O2Cl8(C5H4N4S)(CH3CN), [4] (Black/701.0) |
40.97 (40.51) |
26.67 (27.38) |
01.48 (00.99) |
11.87 (11.98) |
04.97 (04.56) |
09.84 (09.98) |
FTIR Spectra
Table-2 shows that 2-mercaptopyridine35,44-48 hasN-H stretching (3179 cm-1) andS-H stretching (2709 cm-1).
3208 cm-1 peak in [1] implies the presence of N-H functional group. S-H peak at ~ 2708 cm-1 disappears in [1] amounting to thiol group elimination. This further indicates that the ligand has reacted in [1] as thione. C=N bond in [1] has absorbed at the same position as in the free ligand. There is positive shift of C-S frequency to 763 cm-1 due to increase in C-S bond order. Absorption of terminal Mo=O group45-47 (983 cm-1) is observed.
3209 cm-1 peak in [2] implies the presence of N-H functional group. S-H peak at ~ 2709 cm-1 disappears in [2] amounting to thiol group elimination. This further indicates that the ligand has reacted in [2] as thione. C=N bond in [2] has absorbed at 1599 cm-1 showing positive shift in frequency. There is positive shift of C-S frequency to 760 cm-1 due to increase in C-S bond order. Absorption of terminal Mo=O group45-47 (974 cm-1) is observed.
Table 2: shows that 2-Mercaptopyridine35,44-48 hasN-H stretching (3179 cm-1) andS-H stretching (2709 cm-1).
(Infrared frequencies in cm-1) |
|||
Mode |
(2-Mercaptopyridine)35,44-48 |
[1] |
[2] |
υ (N-H) |
3179 |
3208.1 v s |
3209.4 v s |
υ (C-H) |
3054, 2929, 2881 |
3139.1 s, 3079.8 s, 2930.1 m |
3141.4 v s, 3087.4 s, 3023.4 s |
υ (S-H) |
2709 m |
||
υs (C-C ring), ꞷ (C-H Ring) |
1613 s, 1502 s, 1449 s, 1420 s |
1604.5 s, 1529.9 w, 1509.1 m |
1607.3 s |
υ (C=N) |
1585.6 s |
1585.6 s |
1599.3 s |
ρ (C-H ring) |
1248 m, 1188 vs |
1220.2 w |
1225.1 w |
υ (C-S) |
747 |
763.7 v s |
760.2 v s |
Terminal υ (Mo=O)49-51 |
|
983.1 s |
974.4 s |
υs = sym. stretching, ꞷ = out-of-plane bending (wagging),ρ = in-plane bending (rocking)
Table-3 shows N-H stretching peak (3139 cm-1) in [3] of 4-phenylimidazole-2-thiol39,52-54. No S-H stretching in the range 2551-2603 cm-1 in [3] has been observed referring that S-H group has got dissociated. Stretching of C=S (1255 cm-1 and 1225 cm-1) in [3] have been detected. Carbonyl group has greater stretchingwave number than that of thio groupdue to the fact that C=O group is stronger and more polar than C=S group. Intensity of C=O group absorptions is more than that of C=S group. C-S stretching (761 cm-1) has been manifested by [3] owing to origin of Mo-S bond. The latter has stretching at 529 cm-1. Mo=O terminal group stretching (992 cm-1-1010 cm-1) has been reported in literature. This peakwas observed49-51at 968 wave number in [3]. Imidazole-2-thiones39,54 undergoesthiol-thione tautomerism. There is decrease in Mo=O stretching owing to S→Mo coordination39, 55. Mo=O and ligand are trans to each other. It suggests that ligand reacts as thiol during the reaction. Higher wave number of C=N stretching is associated with it.
Table 3: (Infrared frequencies in cm-1)
Mode |
(4-Phenylimidazole-2-thiol)39,52-54 |
[3] |
N-H stretching |
3132, 3247 s |
3139.3 s, 3050.2 s |
S-H stretching |
– |
– |
C=N, C=C stretching |
1561, 1503, 1463 |
1597.0 s, 1508.0 m, 1486.1 m, 1455.6 w |
C=S stretching |
1261, 1111 |
1255.0 m, 1225.2 w, 1102.7 |
C-S stretching |
783 |
761.7 v s |
Mo-S39, 55 stretching |
– |
529.1 w |
Terminal Mo=O49-51 str. |
– |
968.5 s |
6-Mercaptopurine9,56, 57pyrimidine ring stretching (3398 cm-1) in [4] is indicative of N-H group.6-Mercaptopurine9,56, 57imidazole ring N-H stretching is absent, because imidazole ring does not have N-H bondin [4] (Table-4). This supports the presence of Mo-N bond. There is no S-H stretching in [4]. It is concluded that pyrimidine of 6-mercaptopurine reacted as thiol. This fact is also reflected by positive shift of C=N stretching frequency and negative shift of C-S frequency. Here ligand may be acting as multidentate ligand as thione. N→Mo coordination of pyrimidine decreases N-H stretching. Mo-S stretching9,58 has been noticed at 730 cm-1 in [4]. Stretching at 977 cm-1in [4] is concurrent with Mo=O group in terminal position45-47.
Table 4: 6-Mercaptopurine9,56, 57pyrimidine ring stretching (3398 cm-1) in [4]
(Infrared frequencies in cm-1) |
||
Mode |
(6-Mercaptopurine monohydrate)9,56, 57 |
[4] |
N-H Imidazole stretching |
3524 |
|
N-H Pyrimidine stretching |
3376 |
3398.5 v s |
C-H stretching |
3095.0, 2993.8 |
3131.1 v s |
S-H stretching |
2671.5 |
|
C=C stretching |
1669.7 |
1690.0 s |
C=N Imidazole stretching |
1620 |
1622.2 s |
C=N Pyrimidine stretching |
1393 |
1401.9 m |
C-N stretching |
1343.8 |
1311.8 sh |
N-H deformation |
1526.6 |
1520.3 sh |
C=S stretching |
1193 |
1027.9 w |
Mo-S9,58 stretching |
|
730.0 m |
Mo-N9 stretching |
|
562.2 m |
Terminal Mo=O49-51 stretching |
|
977.0 s |
1H NMR Spectra
When 2-mercaptopyridine35,46spectrum is compared with that of [1], it is observed that there is deshielding of protons due to N → Mo coordination (Table-5). N-H resonance occurs at 14.93 δ. C–N bond π electron density increases on coordination.
When 2-mercaptopyridine35,46spectrum is compared with that of [2], it is observed that there is deshielding of protons due to N → Mo coordination (Table-5). There is no N-H peak. C–N bond π electron density increases on coordination. Notations ↓ & ↑ stand for shielding and deshielding.
Table 5: 1H NMR in δ
(1H NMR in δ) |
|||
Protons |
(2-Mercaptopyridine)35,46 |
[1] |
[2] |
N-H |
|
14.93 |
|
C3-H |
7.31 |
7.67 |
7.61 ↓ |
C4-H |
6.82 |
7.28 |
7.23 ↓ |
C5-H |
7.48 |
8.04 |
7.75 ↓ |
NC-H |
7.70 |
8.44 |
8.42 ↓ |
CH3CN |
|
|
1.86 |
N-H chemical shift of 4-phenylimidazole-2-thiol39,59-60 appears at 12.9 δ. There is no particular chemical shift of alcoholic, amino, phenolic and thiolic protons in a solvent, because these protons are labile. There is no existence of N-H and S-H peaks in [3] due to above reasons. There is upfield chemical shift of ring protons and H-5 due to N → Mo coordination.
Table 6: 1H NMR in δ
1H NMR in δ |
||
Protons |
(4-Phenylimidazole-2-thiol)39,59-60 |
[3] |
N-H |
12.98 |
|
S-H |
12.15 |
|
H-5 |
7.52 |
7.39 ↓ |
Aromatic H-2 and H-6 |
8.14 |
7.67 ↓ |
Aromatic H-3 and H-5 |
7.52 |
7.30 ↓ |
Aromatic H-4 |
7.42 |
7.20 ↓ |
6-Mercaptopurine monohydrate57 aromatic N-H absorption (Table-7) is not observed may be due to its participation in bonding. There is no S-H peak may be due to reaction of the ligand as thione. Acetonitrile peak is observed at 2.05 δ.
Table 7: 1H NMR in δ
1H NMR in δ |
||
Protons |
(6-Mercaptopurine monohydrate)57 |
[4] |
N-H (Arom) |
7.20 |
|
N-H |
1.41 |
1.76 ↑ |
S-H |
1.22 |
|
CH3CN |
|
2.05 |
13C NMR Spectra
In 13C NMR, the C=S absorption of 2-mercaptopyridine46, 61-63 in [2] is shifted upfield by 20 ppm (Table-8). This high chemical shift is characteristic of S-bonded thiones. This is due to Mo→S back donation of molybdenum d-orbitals to the sulfur empty π*-orbitals. It increases the C=S π-electron density and shielding of the thiocarbon. C-2 has moved upfield while other carbons have moved downfield. Notations ↓ & ↑ stand for shielding and deshielding.
Table 8: 13C NMR in δ
13C NMR in δ |
||
Carbon |
2-mercaptopyridine46, 61-63 |
[2] |
C=S |
176.57 |
156.40 ↓ |
C-2 |
133.67 |
121.40 ↓ |
C-3 |
136.92 |
137.85 ↑ |
C-4 |
114.12 |
119.01 ↑ |
C-5 (attached to nitrogen) |
138.04 |
149.07 ↑ |
DMSO |
39.5 |
39.42 |
Mass Spectra (LC-MS)64
Ionic species noted (Tables-9, 10) justify the formulae,
![]() |
Table 9: LC-MS Ionization. Click here to View table |
Table 10: LC-MS Ion m/z values
Table 10 (LC-MS Ion m/z values) |
||||
Compound |
Ion |
Calculated64 |
Detected |
Relative intensity |
[1] |
[MoOCl3.2HCl]+ |
290.7 |
293.9 |
22% |
[MoOCl2.2HCl]+ |
255.7 |
258.9 |
4% |
|
[MoOCl2.2HCl]2+ |
127.8 |
129.1 |
6% |
|
[C5H4NS-SNH4C5]+ |
220.1 |
220.0 |
100% |
|
[C5H4NS-NH4C5]+ |
188.0 |
187.0 |
22% |
|
[C5H4N4S]+ |
152.0 |
156.1 |
40% |
|
[C5H4N4S]2+ |
76.0 |
78.1 |
32% |
|
[2] |
[C5H4N4S]+ |
152.0 |
151.0 |
12% |
[C5H4NS-SNH4C5]+ |
220.1 |
221.0 |
100% |
|
[C5H4NS-NH4C5]+ |
188.0 |
189.0 |
32% |
|
[Mo3O2Cl10(CH3CN)2]+ |
757.4 |
759.3 |
5% |
|
[Mo3O2Cl8(CH3CN)2]+ |
687.5 |
684.4 |
7% |
|
[Mo3O2Cl7(CH3CN)]+ |
611.5 |
610.3 |
20% |
|
[Mo3O2Cl6]+ |
535.1 |
536.2 |
17% |
|
[3] |
[Mo2OCl4]+ |
351.6 |
350.9 |
100% |
[C9H8N2S]+ |
176.0 |
177.0 |
35% |
|
[Mo2OCl4(C9H7N2S)]+ |
526.7 |
525.0 |
5% |
|
[Mo2OCl6(C9H7N2S)2]+ |
771.6 |
774.9 |
<1% |
|
[Mo2OCl4(C9H7N2S)2]+ |
701.7 |
701.3 |
<1% |
|
[4] |
[C5H4N4S]+ |
152.0 |
153.0 |
100% |
[Mo2O2Cl6(C5H4N4S)(CH3CN)]+ |
630.6 |
634.0 |
1% |
|
[Mo2O2Cl6(C5H4N4S)]+ |
589.6 |
586.9 |
1% |
|
[Mo2OCl4]+ |
351.6 |
351.0 |
46% |
|
[Mo2O2Cl8(C5H4N4S)(CH3CN)]+ |
700.5 |
699.1 |
1% |
Conclusion
N-H group in [1] absorbs at 3208 cm-1. There is no peak in the region 2709 cm-1, because S-H group has disappeared. There is positive shift of C-S frequency to 763 cm-1 due to increase in C-S bond order confirming the Mo-S bond formation. Ligand has reacted in [1] as thione. Mo=O stretching at 983 cm-1 in [1] confirms that Mo=O is situated at terminal position.
Deshielding of protons shows that there is N → Mo coordination. Ions detected in GC-mass spectrum convince the suggested formula.
[2] shows N-H peak (3209 cm-1). There is no peak in the region 2709 cm-1, because S-H group has disappeared. There is positive shift of C-S frequency to 760 cm-1 due to increase in C-S bond order confirming the Mo-S bond formation. Ligand has reacted in [2] as thione. Mo=O stretching at 974 cm-1 in [2] confirms that Mo=O is situated at terminal position. Ions detected in LC-mass spectrum convince suggested formula. Deshielding of protons shows that there is N → Mo coordination. Presence of CH3CN is confirmed by the presence of 1H NMR peak at 1.86 δ. 13C NMR also justifies the presence of 2-mercaptopyridine.
Thiol group is absent in [3] due to lack of absorptions in the frequency span 2549-2600. Thiocarbonyl group (1255, 1225, 1102 cm-1) exists. C-S stretching (761 cm-1) manifests the origin of Mo-S bond. Mo=O terminal group (968 wave number) is noticed. S-H chemical shift is missing due to absence of thiol group. Ions detected in LC-mass spectrum convince the suggested formula.
S-H is lacking in [4] because of presence of υ(C=S) at 1027 cm-1 and absence of absorption ~ 2672 cm-1. There is no S-H stretching in [4]. Pyrimidine of 6-mercaptopurine reacted as thiol. This fact is also reflected by positive shift of C=N stretching frequency and negative shift of C-S frequency. Here ligand may be acting as multidentate ligand as thione. N→Mo coordination of pyrimidine decreases N-H stretching. There is S→Mo coordination. Mo=O terminal group (977 cm-1) is observed. There is no chemical shift of S-H in 1H NMR of [4]. Presence of CH3CN is confirmed by the presence of peak at 2.05 δ in 1H NMR. Derived formula is supported by LC-mass spectrum.
Acknowledgement
We, the authors express our gratitude to P. U. Chandigarh, India for providing characterizing facility.
Conflict of Interest
There is no conflict of interest among the authors.
References
- https://medlineplus.gov/druginfo/meds/a682653.html.
- https://en.wikipedia.org/wiki/Mercaptopurine#:~:text=Mercaptopurine%20(6%2DMP)%2C,Crohn’s%20disease%2C%20and%20ulcerative%20colitis.
- Łakomska, I.; Pazderski, L.; Sitkowsk, J.; Kozerski, L.; Pełczynska, M.; Nasulewicz, A.; Opolski, A.; Szłyk, E., J. Mol. Struct., 2004, 707, 241-247.
CrossRef - Blank, C.; Dabrowiak, J., J. Inorg. Biochem.,1984, 21, 21-29.
CrossRef - Cuin, A.; Massabni, A. C.; Pereira, G. A.; Leite, C. Q. F.; Pavan, F. R.; Sesti-Costa, R.; Heinrich, T. A.; Costa-Neto, C. M., Biomed. Pharmacother, 2011, 65, 334-338.
CrossRef - Bariyanga, J.; Luyt, A., J. Mol. Struct.,2001, 559, 49-54.
CrossRef - Cini, R.; Cinquantini, A.; Sabat, M.; Marzilli, L. G., Inorg. Chem., 1985, 24, 3903-3908.
CrossRef - Dubler, E.; Gyr, E., Inorg. Chem.,1988, 27, 1466-1473.
CrossRef - Abeer A. Sharfalddin,; Emwas, A. H.; Jaremko, M.; Hussien, M. A., Appl Organomet Chem.,2021, 35, 1-18, DOI: 10.1002/aoc.6041.
CrossRef - Pazderski, L.; Łakomska, I.; Wojtczak, A.; Szłyk, E.; Sitkowski, J.; Kozerski, L.; Kamien´ski, B.; Koz´min´ski, W.; Tousek, J.; Marek, R., J. Molecular Structure, 2006, 785, 205-215.
CrossRef - Murai, T. Chemistry of Thioamides; Springer Nature Singapore Pte Ltd.,2019.
CrossRef - Owczarzak, A. M.; Kubicki, M., Acta Crystallographica, Section E., 2012, E68, o1686. doi:10.1107/S1600536812020090.
CrossRef - Al-R. K. A.; Abdel-Aziz, H. A., Molecules, 2010, 15, 3775-3815.
CrossRef - Borhani, D. W.; Calderwood, D. J.; Frank, K. E. H.; Davis, M.; Josephsohn, N. S.; Skinner, B. S. WO Pat. 2008/063287, 2008.
- Fidanze, S. D.; Erickson, S. A.; Wang, G. T.; Mantei, R.; Clark, R. F.; Sorensen, B. K.; Bamaung, N. Y.; Kovar, P.; Johnson, E. F.; Swinger, K. K.; Stewart, K. D.; Zhang, Q.; Tucker, L. A.; Pappano, W. N.; Wilsbacher, J. L.; Wang, J., Sheppard, G. S.; Bell, R. L.; Davidsen, S. K.; Hubbard, R. D., Bioorg. Med. Chem. Lett., 2010, 20, 2452-2455.
CrossRef - Emmitte, K. A.; Wilson, B. J.; Baum, E. W.; Emerson, H. K.; Kuntz, K. W.; Nailor, K. E.; Salovich, J. M.; Smith, S. C.; Cheung, M.; Gerding, R. M.; Stevens, K. L.; Uehling, D. E.; Jr. Mook, R. A.; Moorthy, G. S.; Dickerson, S. H.; Hassell, A. M.; Leesnitzer, M. A.; Shewchuk, L. M.; Groy, A.; Rowand, J. L.; Anderson, K.; Atkins, C. L.; Yang, J.; Sabbatini, P.; Kumar, R. Bioorg. Med. Chem. Lett., 2009, 19, 1004-1007.
CrossRef - Andreani, A.; Burnelli, S.; Granaiola, M.; Guardigli, M.; Leoni, A.; Locatelli, A.; Morigi, R.; Rambaldi, M.; Rizzoli, M.; Varoli, L.; Roda, A. Eur. J. Med. Chem., 2008, 43, 657-661.
CrossRef - Andreani, A.; Rambaldi, M.; Mascellani, G.; Rugarli, P., Eur. J. Med. Chem. 1987, 22, 19-22.
CrossRef - Amarouch, H., Loiseau, P. R., Bacha, C., Caujolle, R., Payard, M., Loiseau, P. M., Bories, C., Gayral, P., Eur. J. Med. Chem., 1987, 22, 463-466.
CrossRef - Gupta, G. D., Jain, K. K., Gupta, R. P., Pujari, H. K., Indian J. Chem., Sect. B: Org. Chem. Incl. Med. Chem., 1983, 22, 268.
CrossRef - Vu, C. B.; Bemis, J. E.; Disch, J. S.; Ng, P. Y.; Nunes, J. J.; Milne, J. C.; Carney, D. P.; Lynch, A. V.; Smith, J. J.; Lavu, S.; Lambert, P. D.; Gagne, D. J.; Jirousek, M. R.; Schenk, S.; Olefsky, J. M.; Perni, R. B., J. Med. Chem., 2009, 52, 1275-1283.
CrossRef - Andreani, A.; Rambaldi, M.; Andreani, F.; Bossa, R.; Galatulas, I., Eur. J. Med. Chem. 1988, 23, 385-389.
CrossRef - Andreani, A.; Rambaldi, M.; Locatelli, A.; Bossa, R.; Fraccari, A.; Galatulas, I., Pharm. Acta Helv., 1993, 68, 21-24.
CrossRef - Andreani, A.; Bonazzi, D.; Rambaldi, M., Arch. Pharm., 1982, 315, 451-456.
CrossRef - Andreani, A.; Rambaldi, M. M.; Locatelli, A.; Bossa, R.; Fraccari, A.; Galatulas, I., J. Med. Chem., 1992, 35, 4634-4637.
CrossRef - Ding, H.; Chen, Z.; Zhang, C.; Xin, T.; Wang, Y.; Song, H.; Jiang, Y.; Chen, Y. Xu, Y.; Tan, C. Molecules, 2012, 17, 4703-4716.
CrossRef - Abdelwareth Sarhan, A.O.; Al-Dhfyan, A.; Al-Mozaini, M. A.; Adra, C. N.; Aboul-Fadl, T. Eur. J. Med. Chem., 2010, 45, 2689-2694.
CrossRef - Poorrajab, F.; Ardestani, S. K.; Emami, S.; Behrouzi-Fardmoghaddam, M.; Shafiee, A.; Foroumadi, A. Eur. J. Med. Chem., 2009, 44, 1758-1762.
CrossRef - Khalaj, A., Nakhjiri, M., Negahbani, A. S., Samadizadeh, M., Firoozpour, L., Rajabalian, S., Samadi, N., Faramarzi, M. A., Adipour, N., Shafiee, A., Foroumadi, A., Eur. J. Med. Chem., 2011, 46, 65-70.
CrossRef - Kumaresan, K. L. Lu, S.; Wen, Y. S. ; Hwu, J. R., Organometallics, 1994, 13, 3170–3176.
CrossRef - Nagai, K.; Carter, B. J.; Xu, J.; Hecht, S. M., J. Am. Chem. Soc., 1991, 113, 5099-5100.
CrossRef - Lobana, T. S.; Bhatia, P. K., J. Sci. Ind. Res., 1989, 48, 394-401.
- Singh, G.; Mangla, V.; Goyal, M.; Singla, K.; Rani, D., American International Journal of Research in Science, Technology, Engineering & Mathematics, 2014, 8(2), 131-136.
- Singh, G.; Mangla, V.; Goyal, M.; Singla, K.; Rani, D., American International Journal of Research in Science, Technology, Engineering & Mathematics, 2015,9(1), 25-33.
- Singh, G.; Mangla, V.; Goyal, M.; Singla, K.; Rani, D., American International Journal of Research in Science, Technology, Engineering & Mathematics, 2015,10(4), 299-308.
- Singh, G.; Mangla, V.; Goyal, M.; Singla, K.; Rani, D., International Congress on Chemical, Biological and Environmental Sciences,2015, 930-942, May 7-9, Kyoto (Japan).
- Singh, G.; Mangla, V.; Goyal, M.; Singla, K.; Rani, D.; Kumar, R., American International Journal of Research in Science, Technology, Engineering & Mathematics, 2016, 16(1), 56-64.
- Singh, G.; Kumar, R., American International Journal of Research in Science, Technology, Engineering & Mathematics, 2018, 22(1), 01-08.
- Rani, D.; Singh, G.; Sharma, S., Oriental Journal of Chemistry, 2020, 36(6), 1096-1102.
CrossRef - Rani, D.; Singh, G.; Sharma, S., Oriental Journal of Chemistry, 2021, 37(1), 46-52.
CrossRef - Mangla, V.; Singh, G., American International Journal of Research in Science, Technology, Engineering & Mathematics, 2019,26(1), 145-148.
- Mangla, V.; Singh, G., Oriental Journal of Chemistry, 2019, 35(3), 1094-1102.
CrossRef - Vogel, A. I., A Text Book of Quantitative Inorganic Analysis; John Wiley and Sons: New York, 1963 (Standard methods).
- http://www.sigmaaldrich.com/catalog/product/aldrich/m5852?lang=en®ion=IN.
- Refat, M. S.; Farias, R. F. D., J. Serb. Chem. Soc., 2006, 71(12), 1289-1300.
CrossRef - Hanif, M.; Saddiq, A.; Hasnain, S.; Ahmad, S.; Rabbani, G.; Isab, A. A., Spectroscopy, 2008, 22, 51-56.
CrossRef - Xhang, H. L.; Evans, S. D.; Henderson, J. R.; Miles, R. E.; Shen, T., J. Phys. Chem. B, 2003, 107, 6087-6095.
CrossRef - Shpakovsky, D. B.; Banti, C. N.; Houle, G. B.; Kourkoumelis, N.; Manoli, M.; Manos, M. J.; Tasiopoulos, A. J.; Hadjikakou, S. K.; Milaeva, E. R.; Charalabopoulos, K.; Bakas, T.; Butlerd, I. S.; Hadjiliadisa, N., Dalton Trans., 2012, 41, 14568-14582.
CrossRef - Barraclough, C. G.; Kew, D. J., Australian J. Chem., 1970, 23, 2387-2396.
CrossRef - Ward, B. G.; Stafford, F. E., Inorg. Chem.,1968, 7, 2569.
CrossRef - Bodo, H. H.; Regina, Z. Chem., 1976, 16, 407.
CrossRef - Jolley, J.; Cross, W. I.; Pritchard, R. G.; McAuliffe,C. A.; Nolan, K. B., Inorganica Chimica Acta, 2001, 315, 36-43.
CrossRef - Kahn, E. S.; Rheingold, A. L.; Shupack, S. I., J. Crystallographic and Spectroscopic Research, 1993, 23 (9), 697-710.
CrossRef - Trzhtsinskaya, B. V.; Abramova, N. D., J. Sulphur Chemistry, 1991, 10(4), 389-430.
CrossRef - Abramenko, V. L.; Sergienko, V. S., Russian J. Inorg. Chem., 2009, 54(13), 2031-2053.
CrossRef - Kumar, G. P.; Sanganal, J. S.; Phani, A. R.; Tripathi, S. M.; Manohara, C.; Raghavendra, H. L.; Janardhana, P. B.; Amaresha, S.; Swamy, K. B.; Prasad, R. G. S. V., Phamacological Research, 2015, 100, 47-57.
CrossRef - http://www.molbase.com/en/hnmr_6857-34-7-moldata-838140.html#tabs.
- Ueyama, N.; Nakata, M.; Araki, T.; Nakamura, A., Chemical Soc. Japan, Chemistry Lett., 1979, 421-424.
CrossRef - Abramenko, V. L.; Sergienko,V. S.; Churakov, A. V., Russian J. Coord. Chem., 2000,26(12), 866-871.
CrossRef - Sharma, M.; Koty, A.; Srivastava, M.; Srivastava, A., J. Chinese Chemical Society, 2007, 54, 1419-1432.
CrossRef - https://spectrabase.com/spectrum/1wIN1BStPn8.
- https://www.chemicalbook.com/SpectrumEN_2637-34-5_13CNMR.htm.
- https://pubchem.ncbi.nlm.nih.gov/compound/2-
Mercaptopyridine#section=13C-NMR-Spectra. - http://www.sisweb.com/referenc/tools/exactmass.html.
This work is licensed under a Creative Commons Attribution 4.0 International License.