Adsorptive Removal of Chromium (VI) Using Silver Nanoparticles Synthesized Via Green Approach with the Extract of Moringastenopetala
1Department of chemistry,Dilla University, College of Natural and Computational Sciences,P. O. Box 419, Dilla, Ethiopia.
2Dilla University, University Industry Linkage Coordinator, P. O. Box 419, Dilla, Ethiopia.
3Dilla University, Reform office coordinator, P.o.Box 419, Dilla, Ethiopia
.
Corresponding Author E-mail: tagesse99@gmail.com
DOI : http://dx.doi.org/10.13005/ojc/370217
Article Received on : 27-Dec-2020
Article Accepted on : 11-Apr-2021
Article Published : 07 Apr 2021
In the current study, the potential sorption of Cr (VI) ions through a zero-valent silver nanoparticle synthesized by a green approach has been studied. The objective of this research was to remove Cr (VI) from aqueous solution using zero-valent silver nanoparticles synthesized from Moringa stenopetala leaves extract. The synthesized silver nanoparticles were characterized using X-ray diffractometer and UV-visible spectrophotometer. The grain size of nanoparticles was found to be 19.5 nm confirming the crystalline structure of the mesosphere and showed the maximum absorption at 419 nm. In order to determine parameters that influence the adsorption mechanism, batch adsorption experiments have been conducted. Results obtained for removal of Cr (VI) ions showed that efficiency of removal improved as adsorbent dosage increased until the equilibrium point reached at 50 minutes. However, as the pH of the solution increased from 1 to 8, and as the Preliminary concentration of Cr increased, removal efficiency decreased. Adsorption isotherms were investigated by applying the models of Langmuir and Freundlich. The Freundlich isotherm can better explain the removal of Cr (VI) ions, offering correlation coefficient values greater than the Langmuir isotherm model. Adsorption kinetics regression results studied by the pseudo-first and second-order models moreover proved that a pseudo-first-order was further accurately represented by the adsorption kinetics. In order to alleviate wastewater problems, the implementation of this approach is able to cost-effective, eco-friendly and publicly viable. To improve its removal effectiveness and compare it with other adsorbents, additional study on surface modification of this adsorbent is required.
KEYWORDS:Adsorption Isotherm; Green Synthesis; Hexavalent Chromium; Kinetics; Moringa Stenopetala; Silver Nanoparticle
Download this article as:
Copy the following to cite this article: Tagesse W, Haile B. Adsorptive Removal of Chromium (VI) Using Silver Nanoparticles Synthesized Via Green Approach with the Extract of Moringastenopetala. Orient J Chem 2021;37(2). |
Copy the following to cite this URL: Tagesse W, Haile B. Adsorptive Removal of Chromium (VI) Using Silver Nanoparticles Synthesized Via Green Approach with the Extract of Moringastenopetala. Orient J Chem 2021;37(2). Available from: https://bit.ly/39OUdbm |
Introduction
Background of the study
Nanotechnology is a technology that is one of the world’s most innovative approaches. Nanotechnology describes a number of nanometer-scale technologies that range from individual atoms or molecules to around 100 nanometers. The noble metal nanoparticles such as Ag, Au, Pd, and Pt are the focus of attention today because of their strong optical absorption in the visible region caused by the collective excitation of free-electron gas.2 Biosynthesis of nanoparticle is eco-friendly, time-efficient and cost-effective. More significantly, the biosynthesized NPs on their surface are free of toxic materials. Green production of AgNPs with biological extract is a healthy as well as advantageous way to synthesize metallic nanoparticle since it is readily accessible. In addition, plant extract can serve as both a reducing and stabilizing agent during the synthesis of AgNPs. Photochemicals such as flavonodis, terpenodis, terpenes, flavones, phenolics, polysaccharides, saponins, tannins and alkaloids have been used for the synthesis of AgNPs. 3These photochemicals contain hydroxyl, aldehyde, ketone, carboxyl, and amino functional groups capable of reducing Ag+ ions 3
Nanomaterials have been under intensive study over the last decade and effectively implemented in several areas such as, catalyst fabrication, medication, artificial intelligence, and life science. Due to large specific surface areas, nanomaterials show high adsorption abilities, reactivity, and freely move in solution. It has been documented that heavy metals, organic contaminants, inorganic anions, and bacteria are successfully removed by different kinds of nanomaterials. Nanomaterials demonstrate great assurance for practical use in water-based and industrial effluent treatment on basis of frequent studies. [4] Toxic metals like Cu, Pb, Zn, Ni, Hg, Cr, etc present in the effluents of chemical industries like finishing and electro-plating, mining and tanneries are among the chief pollutants of surface and groundwater which harm both the fauna and flora in aquatic systems and are detrimental to human health. Relatively costy techniques including exchange adsorption, solvent extraction and filtration have been used for removal of heavy metals. A benefit of the adsorption approach is cost effective and recyclable. 5, 6
Nowadays, zero-valent nanoparticles of metals, nanoparticles of metal oxides, carbon nanotubes (CNTs), and nanocomposites are widely researched nanomaterials for water and wastewater treatment. However, the objective of this study is to evaluate the removal potential of zero-valent silver nanoparticles synthesized from leaves of Moringa stenopetala (MS) as mediator for the removal of hexavalent Chromium from aqueous solution. No literature data are available on removal of Cr (VI) ions by silver nanoparticle synthesized with Moringa stenopetala leaves.
Experimental Part
Moringa stenopetala leaves Collection
Moringa stenopetala leaves from the Dilla University compound, Ethiopia, were collected. To remove any particulate matter, the collected leaves were thoroughly washed under tap water and then washed with distilled water. The leaves were then oven dried at 50 °C and cut into tiny pieces.
Solvent Extraction
Moringa stenopetala extract was prepared by boiling 20g Moringa stenopetalaleaf at 60 oC in 0.1L of distilled water for 5 min. The mixture was then filtered and the filtrate was kept at 4 °C until used as reducing and capping agent during the synthesis of Ag nanoparticles.
Green synthesis of AgNPs
The synthesis of AgNPs was performed by the method of bottom-up approaches starting with a precursor of metal salt (dissolved in a solvent) that is reduced in a chemical reaction and the NPs are formed by a nucleation process followed by a cluster growth. 7 2 mL of Moringa Stenopetala leaves extract was taken for the synthesis of zerovalent silver nanoparticles and added to 20 mL of AgNO3 (1 mM). The color of the solution changed to dark brown. Finally, the solution was stirred for 40 min followed by centrifuging for 20 minutes at 150 rpm and washed five times to remove residues of Ag+ ions. This experiment was performed at room temperature. The presence of the dark brown color confirmed the production of Ag nanoparticles. The centrifuged Ag nanoparticles were then used for further experiment. 8
Preparation of working standard solutions
Cr(VI) stock solution (1000 mg/L) was first prepared from potassium dichromate (99.9 percent, BDH) by dissolving 2.28 g k2Cr2O7 in deionized water, thoroughly mixed and the solution was diluted to the mark of 1 L standard bottle. An intermediate solution of 100 ppm hexavalent chromium was then prepared by taking 50 mL of the stock solution and diluting it through deionized water into 500 mL standard flask. For method calibration, six working standard solutions with different concentrations of 1.0, 3.0, 5.0, 7.0, 9.0 and 11 ppm were prepared. (Fig. 1).
![]() |
Figure 1: Method calibration curve. |
Removal experiments
The batch experiments were carried out by adding 30 mL of Cr(VI) solution into 250 mL standard flasks at the desired adsorbent dose, pH, and contact time at room temperature. The solutions were shaken using electronic mechanical shaker for a definite period of time. Effect of adsorbent dose (25 to 125 mg), contact time (5 to 60 min), pH (1 to 8), initial concentration of adsorbate (1, 3, 5, 7, 9 and 11 mg/L) were studied by continuous variation method at 120 rpm agitation speed. The pH of the Cr(VI) containing solution was adjusted using 1×10-1 mol L-1 hydrochloric acid or 1×10-1 mol L-1 sodium hydroxide.
The Cr (VI) concentrations in the test solutions were controlled by UV-Vis-spectrometer at 540 nm. Experiments were carried out in two parameter options to confirm the observed effects instead of taking triplicate measurements. The percentage of the removal of Cr (VI) from aqueous solution was estimated using the formula: 6

Evaluation of adsorption efficiency
Adsorption isotherm is very interesting to consider and describe how the solutes interact with the adsorbent. To illustrate the removal process, the adsorption data were fitted to the two commonly used isothermmodels namely: Langmuir and Freundlichgiven in equation (3) and (4) respectively. Lagergren’s pseudo first-order model in equation (5) and pseudo-second-order model in equation (6) were used to fit the kinetic adsorption data, and all of the kinetic parameters for chromium (VI) ion were determined from their graphs. The amounts of hexavalent chromium adsorbed on the Ag nanoparticles were represented by the Adsorption capacity (q, mg/g) and calculated as shown in equation (2):

Where m is adsorbent mass expressed by g, V solution volume in L and Co and Ce are the original and equilibrium Cr (VI) amounts, expressed by mg L-1, respectively. Using the statistical applications Origin pro 8 and Microsoft excel 2007, plots and linear regression were performed. Recorded data from linear regression contains coefficient value of R2 correlation.9
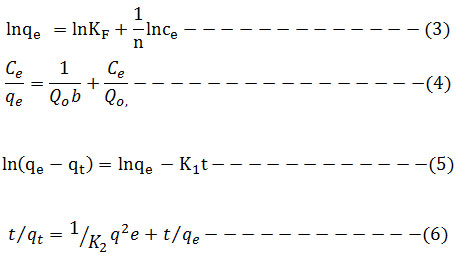
Characterization of Adsorbent
X-ray diffractometer analysis
X-ray diffractometer (XRD) with 2θ scope of 10-800 using CuKα X-ray source was applied to study the crystal structure and amorphous nature of the prepared zero valent silver nanoparticle. The prepared powder of AgNPs was filled into a sample holder of aluminum. X-ray diffraction analysis of the samples was carried out on a 40 kV and 30 mA working diffractometer with a Cu-Ka radiation wavelength of Lambda = 1.5406 Å. Using Origin 8.0 software, the XRD spectra were analyzed, and the crystallite particle size was determined from the width of the peaks by taking the maximum strong pick, using Scherrer’s equation formula (7).10

UV-Vis Spectroscopy
The chemical reduction of monovalent silver to zero valent silver metal was controlled by determining the UV-Vis absorption spectrum of the solution containing a small aliquot of the sample in deionized water kept for four hours.11
Results and Discussions
Visual observation
The reduction of Ag+ ion into Ag0 metal nanoparticle upon exposure to Moringa Stenopetala leaf extract resulted color change. The yellowish green suspension of Moringa Stenopetala leaf extract (Fig. 2A) turned into dark brown emulsion (Fig. 2C) after the addition of AgNO3 (Fig. 2B) and 24 hours continuous stirring at room temperature.
![]() |
Figure 2: (A) Yellowish green extract of M. stenopetela leaves. (B) Color less AgNO3 solution. (C) Dark brown emulsion. |
Surface-Plasmon Resonance is responsible for the color changes in aqueous solution (Fig. 2). Synthesis mechanism of AgNPs can vary with nature of phytochemicals. However, the chief mechanism is the reduction of Ag+ ions by specific functional groups. During preparation of AgNPs the possible mechanism is silver ions dispersed into the extract of Moringa stenopetala leaf after that the reaction takes place to form [Ag (Moringa stenopetala leaf extract) + complex]. In the extract there is aldehyde functional group that is used to reduce silver mono valent to silver zero valent. Therefore, AgNPs is formed while aldehyde functional group oxidized to carboxylic acid groups. The carboxylic acid groups are left in the solution but AgNPs is precipitated in the test tube, which is in agreement with Smaranika et al., 12
Characterization of nanoparticles
X-ray diffractometer characterization
Figure 3 demonstrated the XRD blueprint of Ag nanoparticle formed. The synthesized silver nanoparticle has the characteristic peaks on the pattern of AgNPs at 2θ values 34.85⁰, 38.52⁰ and 44.77⁰conforms to lattice plane at (111) and (200) of metallic silver respectively as shown in (Fig. 3). Moreover, X-ray diffractometer blueprint confirms face centered cubic structure of metallic silver and the data is correlated with international center for diffraction data (ICDD PDF2). The additional peaks at 2θ values can be approved due to the organic residues of the plant extract, which is in agreement with Saba et al.,13.According to the Scherer calculation, the grain size of the synthesized AgNPs obtained is 19.5 nm resulting using peak FWHM, which is matching to 111 planes. Different research works show that green synthesized NPs with particle sizes below 20 nm are highly reactive.9
![]() |
Figure 3: XRD pattern of synthesized silver nanoparticle powder. |
UV-Vis Spectroscopy
The maximum absorption peak at 419 nm (Fig. 4A) is due to Surface Plasmon Resonance that point toward the AgNPs formation. Silver nanoparticles have free electrons so as to give the Surface Plasmon Resonance absorption band. A single Surface Plasmon Resonance band is revealed in current study that proves no agglomeration of AgNPs. It is observed that the absorption of silver nanoparticles increases as the incubation time increases to 24 hr (Fig. 4B). The maximum attainability in the stability of AgNPs and constant absorption after at 24 hr reaction time is observed. Abambagade and Belete have previously recorded similar outcomes. 14
![]() |
Figure 4: (A) UV-Vis absorption spectrum of AgNPs synthesized (B) Absorbance recorded as function of incubation period |
Influence of PH
A potential of AgNPs to remove hexavalent chromium in aqueous solution at different pH values (1 – 8) is shown in (Fig. 5). All other parameters were kept constant: contact time (60 min), dosage (0.05 g) and the initial metals concentration (5.5, 11 mg L-1). Adsorption of hexavalent chromium ion was maximum at lower pH and rapidly decreases as pH values rise up to 8. Chromium (VI) can occur primarily like CrO4-2, Cr2O7-2, HCrO4–, H2CrO4 and H2Cr2O7 in aqueous solutions. CrO4-2 predominates at high pH while hydrogen chromate (HCrO4–) predominates at low pH. The HCrO4– form is thus inferred at low pH is the main type of hexavalent chromium favorable sorbed by AgNPs. Because of the addition of acid H+, the surface of the adsorbent is positively charged ions at low pH and this facilitates the binding of negatively charged HCrO4– ions. Thus, with decreasing pH, the adsorption of hexavalent chromium ions enhances noticeably. When the pH is increase, AgNPs losing surface positivity and these results in a dropping in electrostatic interaction force between adsorbent and adsorbate ion. 15
![]() |
Figure 5: pH optimization. |
Influence of initial concentration of hexavalent Chromium
Influence of initial concentration on hexavalent Chromium removal by AgNPs was studied at various initial concentrations ranging 1 to 11 mg L-1 at pH 1, contact times (60 min) and at different adsorbent dose (50 mg and 25 mg). The percentage removal depends primarily on the initial metal concentrations. Hexavalent chromium ions removal at different concentration as shown in (Fig. 6) that the removal percentages decrease with increases in metal ions concentrations.
Removal efficiency of 97% has been achieved using a 1ppm initial Cr(VI) concentration. The decrease in percentage removal with an increase in initial concentration may be due to lack of enough surface area to accommodate much more Cr(VI) ions accessible in the solution. This explanation may be supported by the knowledge that at extremely low hexavalent chromium ions, the ratio of the available surface of the adsorbents to the initial concentration of chromium is high, creating a higher chance of chromium removal.Binding sites get quickly saturated when increasing in initial chromium concentration as adsorbent dose remained constant. Hence, in case of high adsorbate concentrations the percentage removal decreases. 16
![]() |
Figure 6: Influence of initial concentration adsorption. |
Influence of contact time
The influence of time in Cr(VI) reduction was studied for hexavalent Chromium concentration at 5.5 x 103 and 11x103 μg L-1 , pH 1, 0.05 g adsorbent dose, 120 rpm stirring speed and different contact times (10-60 min), respectively. (Fig. 7). The results showed that highest percentage removal of Cr(VI) has been achieved at 50 min.. This indicates that 50 min was required to reach equilibrium for Cr (VI) removal on AgNPs. As contact time increased, no significant increment in hexavalent Chromium ion reduction from the solution was noticed. Therefore, mass transfer from a mass solution to binding sites is affected by contact time. 9
![]() |
Figure 7: The influence of contact time on hexavalent chromium adsorption. |
Influence of dosage
The influence of the adsorbent dose in the removal of hexavalent chromium ions should be investigated. The effect of adsorbent mass (25-125 mg) on removal of hexavalent chromium at initial Cr(VI) concentrations (5.5 ppm and 11 ppm), at pH 1,and at 50 minutes of contact time was studied.The results, represented by (Fig. 8), indicated that when the amount of AgNPs increases, the hexavalent chromium removal efficiency enhances and the removal efficiency became constant at higher amounts of AgNPs. The direct relationship between adsorbent dose and adsorption efficiency is because of the fact that more exchangeable sites for chromium ions and greater surface area of AgNPs are accessible when dose of adsorbent in solution is high.17
![]() |
Figure 8: Influence of dosage on adsorption |
Adsorption isotherm study
The functional relation between the concentration of hexavalent chromium ions sorbed on the AgNPs surface and hexavalent chromium ions left in solution was tested with Langmuir and Freundlich adsorption isotherms. Further Langmuir isotherm analysis is able to done using the separation factor (RL) which is unit less immeasurable extent. The RL value for a favorable adsorption lies between 0 and 1 while RL value exceeds1 for unfavorable adsorption, Adsorption is linear for RL = 1 and it is irreversible if RL = 0. In this analysis, the separation factor RL is 0.844, which explains the favorable adsorption process for removal of Cr (VI).The constants of Freundlich; kf and n cab are determined by plotting the graph between lnqe and lnCe as shown in (Fig. 10). High n value (2.1) indicates that Ag nanoparticles are better adsorbents for aqueous solution intake of Cr (VI). The Langmuir equation in the Ce/qe versus Ce graph is seen in (Fig. 9). The values are obtained as 5.435 mg g−1 at room temperature. The linear relationship for the Freundlich isotherm model is higher than for the Langmuir isotherm model. (tables 1 and 2 ). 18
![]() |
Figure 9: Langmuir model |
![]() |
Figure 10: Freundlich model. |
Table 1: Adsorption isotherm data.
Absorbance |
Ce |
Qe |
Ce/qe |
Lnqe |
Ln ce |
0.0276 |
0.03421 |
0.5795 |
0.059 |
-0.55 |
-3.4 |
0.037 |
0.282 |
1.63 |
0.173 |
0.488 |
-1.3 |
0.049 |
0.5974 |
2.642 |
0.226 |
0.972 |
-0.52 |
0.078 |
1.361 |
3.384 |
0.4022 |
1.22 |
0.31 |
0.104 |
2.045 |
4.173 |
0.49 |
1.43 |
0.72 |
0.139 |
2.966 |
4.821 |
0.62 |
1.6 |
1.1 |
Table 2: Adsorption isotherm parameters for Cr(VI) removal from synthetic effluent by AgNPs.
Langmuir Freundlich |
|||||||
Metal |
(mgg-1) |
b(g mg-1) |
RL |
R2 |
n |
Kf (Lg-1) |
R2 |
Cr(VI) |
5.435 |
0.034 |
0.844 |
0.972 |
2.1 |
3.021 |
0.995 |
Kinetic study
Pseudo first and Pseudo – second order kinetic models were used to study Cr (VI) sorption on the surface of AgNPs. (Figs. 11 and 12) illustrate experimental data plots at different contact time for pseudo first and second order models, respectively. The Pseudo first order considers that the speed at which adsorption sites are occupied is directly dependent on the number of unoccupied sites.19 The Pseudo Second Order model considers that the square of number of vacant sites on the adsorbent is affected by the speed of adsorption in metal ions. The higher values of the correlation coefficients (R2) and the comparative analysis of the observational adsorption capacity, qe(exp) with the measured value, qe(cal) acquired for the removal of Cr(VI) ions indicated that the pseudo-first order model was excellent than the pseudo-Second order. (table 3 below) 20
![]() |
Figure 11: Pseudo first order kinetic. Click here to View figure |
![]() |
Figure 12: Pseudo-second-order kinetic. |
Table 3: Pseudo- first and second- order kinetics for sorption of hexavalent chromium onto AgNPs
Pseudo first-order |
Pseudo second-order |
qe(exp) 6.908 |
qe(exp) 6.908 |
qe(cal) 7.1627 |
qe(cal) 5.294 |
K1 0.1261 |
K2 0.0052 |
R2 0.9936 |
R2 0.7879 |
Conclusion
In the current study one pot method for the production of AgNPs using Moringa stenopetala leaves extract has been reported. This approach is very quick, appropriate, less time-consuming, and environmentally friendly and can be used in a number of current applications. The green synthesis of Ag nanopartilces using Moringa stenopetala leave extract was successful which is mesosphere’s crystalline structure having 19.5 nm particle size. The experimental results indicated that AgNPs were effective in water purification and environmental remediation. Parameters like influence of pH, influence of contact time, influence of dosage and influence of hexavalent chromium concentration were optimized. Among these parameters, the most significant parameter that influences the sorption methods was established to be pH optimization. Two isothermal models Langmuir and Freundlich were used to model the results obtained. An equilibrium isotherm was well described by Freundlich equation, which showed that the surface is heterogeneous with n values 2.1 capable of multilayer adsorption and therefore favorable adsorption has occurred. The selected adsorbent has removed 97 % Cr (VI) from the aqueous solution. The pseudo-first-order model equation can well explain the adsorption kinetics, since R2 is greater than 0.99 at low concentration, but the pseudo-second-order model equation shows R2 = 0.78. In order to improve its removal effectiveness and compare it with other adsorbents, yet more experimentation on the chemical modifications of this adsorbent is needed.
Acknowledgment
The authors would like to thank the Dilla University College of Natural and Computational Sciences Department of Chemistry for the tools and chemicals provided.
Conflict of interest
No conflict of interest was declared by the authors.
Funding Sourse
The authors received no direct funding for this research.
Reference
- Kanchi, S. Environmental Analytical Chemistry, 2014, 1(2), 1-3. https://DOI: 10.4172/2380-2391.1000e102
CrossRef - Davoud, D.; Shiva, T.; Farzane, K. Soft Nanoscience Letters,2013,3 (4), 93-100. DOI: 10.4236/snl.2013.34017
CrossRef - Arul Dhas, N.; Paul Raj, C.;Gedanken, A. Chem. Mater, 2018, 10(5), 1446–1452. https://doi.org/10.1021/cm9708269
CrossRef - Shakeel, A.; Mudasir, A.; Babu Lal, S.; Saiqa, I. Journal of Advanced Research20167(1), 17–28https://doi.org/10.1016/j.jare.2015.02.007
CrossRef - Haijiao, L.; Jingkang, W.; Marco, S.;Ting, W.; Ying, B.; Hongxun, H. Advances in Materials Science and Engineering.2016, 3 https://doi.org/10.1155/2016/4964828
CrossRef - Burks, T. kth royal institute of technology school of chemical science and engineering, 2016,4, 1-76. urn:nbn:se:kth:diva-179871
- Rauf ,M. A.; Iqbal , M. J.; Ikram , M.; Rauf, N. Adsorption Studies of Ni(II) from Aqueous Solution onto Bentonite. Soil and environmental sciences, 2003, 21(2). https://doi.org/10.1081/TMA-120020267
CrossRef - Ibrahim, M.; Ehab, S.Scrivener Publishing LLC2018, 293-319. Book chapter 10 https://doi.org/10.1002/9781119418900.ch10
CrossRef - Khairia, M.; Al-Qahtani. Egyptian Journal of Aquatic Research, 2017, 43(4), 269-273. https://doi.org/10.1016/j.ejar.2017.10.003
CrossRef - Workineh, M. F.; Wendimagegn, T.D.; Girmaye, Benti. Cogent Food & Agriculture, 2020, 6,1-14 https://doi.org/10. 1080/23311932 .2020. 1816420
CrossRef - Prasad , TNVKV.; Elumalai, EK. Asian Pacific Journal of Tropical Biomedicine, 2011, 1(6), 439-442. doi: 10.1016/S2221-1691(11)60096-8
CrossRef - Smaranika, D.; Umesh, K.; Birendra, K.International Journal of Nanotechnology and Application, 2013, 3 (2), 51-62.
- Saba, A.; Hussein, R. International Journal of ChemTech Research, 2017, 10 (3), 508-514. https://www.researchgate.net/publication/316147987
- Abambagade, A.; Belete, Y.African Journal of Biotechnology, 2017, 16 (32), 1705- 1716. https://doi.org/10.5897/AJB2017.16010
CrossRef - Khairia, M.; Al-Qahtani.American-Eurasian Journal of Toxicological Sciences, 2016, 8, 102-109. https://doi.org/10.5829/ idosi.aejts. 2016.8.2.10422
- Mohamed, E.; Fatima, Al-T.; Ragwan, M.; Khadija, A.American Journal of Analytical Chemistry, 2015, 6, 3. DOI: 10.4236/ajac.2015.61001
CrossRef - Tolaymat, T.M.; El Badawy, A.M.; Genaidy, A.; Scheckel, K.G.; Luxton, T.P.; Suidan, M. Sci. Total Environ., 2010, 408 (5), 999–1006. DOI: 10.1016/j.scitotenv.2009.11.003
CrossRef - Radia, L.; Oumessaad, B.; Adh’ Ya, E.; Andre, ́D. Adsorption Science & Technology, 2018, 36(3-4). https://doi.org/10.1177/0263617417750739
CrossRef - Thilagan, J.; Gopalakrishnan, S.; Kannadasan, T. International Journal of Pharmaceutical and Chemical Sciences, 2013, 2(2), 1062. https://www.researchgate.net/publication/288617367
- Hojat, V.; Sirous, A. Pourya, M. Journal of Cleaner Production,2018,170, 1536-1543.https://doi.org/10.1016/j.jclepro.2017.09.265.
CrossRef
This work is licensed under a Creative Commons Attribution 4.0 International License.