A Comparative Study of Photo-sensitizers in Reductant Surfactant System in Photogalvanic cell for Photo induced Power Generation and Storage
Photo Electrochemistry Lab, Department of chemistry, New Campus, Jai Narain Vyas University, Jodhpur (Rajasthan), India.
Corresponding Author E-mail: rathorejayshree29@gmail.com
DOI : http://dx.doi.org/10.13005/ojc/400211
Article Received on : 02 Dec 2023
Article Accepted on :
Article Published : 11 Mar 2024
Reviewed by: Dr. Pooran Koli
Second Review by: Dr. Narpat Raj Nenival
Final Approval by: Dr. Tanay Pramanik
In the recent research we compared the solar energy performance of photogalvanic cell (PG cell) for two food dyes that act as photosensitizer tatrazine and sunset yellow in the DSS-EDTA (Dioctyl sodium sulphosuccinate - Ethylenediamine tetraacetic acid) system for power generation and its storage. Both are azo food dyes and act as photosensitizer, DSS acts as an anionic Surfactant and EDTA act as a reductant. The electrical parameters of both dye systems were studied and compared. The observed conversion efficiencies and fill factor for both systems in the DSS-EDTA system were 0.6163%, 0.2800, and 1.2186%, 0.2900, respectively. The stable photopotential (Voc) was 879 mV and 864.0 mV whereas the stable photocurrent (isc) was 220µA and 390 µA, respectively. In addition to having built-in storage capacity, the photogalvanic cell can operate in dark conditions. The amount of time required for the cell to reach half the value of power at power point, which was measure its performance called t1/2 of the cell. Cell performance of both PG cell was determined and for the Tartrazine - EDTA - DSS system it has been obtained 100 minutes while for the sunset yellow - EDTA - DSS system it was observed at 140 minutes. The current-voltage (i-v) characteristics of both the systems have been studied.
KEYWORDS:Current-voltage; Dye; Photosensitizer; Photopotential; Photocurrent; Reductant
Download this article as:
Copy the following to cite this article: Rathore J, Arya R. K. A Comparative Study of Photo-sensitizers in Reductant Surfactant System in Photogalvanic cell for Photo induced Power Generation and Storage. Orient J Chem 2024;40(2). |
Copy the following to cite this URL: Rathore J, Arya R. K. A Comparative Study of Photo-sensitizers in Reductant Surfactant System in Photogalvanic cell for Photo induced Power Generation and Storage. Orient J Chem 2024;40(2). Available from: https://bit.ly/3IsqYMu |
Introduction
Solar energy is the most promising solution of all our environmental and energy issues. Solar energy free of cost, nonpolluting and mother of whole energy sources on earth. Solar energy is more capable source for sustainability than fossil fuel as we can use solar energy without diminishing its future availability. Goals like energy security, mitigation to climate change of society can be possible by using solar energy. There is another name for the photogalvanic cells: liquid-junction solar cells. The PG cells are light-harvesting devices that convert solar energy directly into electrical energy. They have an internal storage capacity and are regulated by diffusion, meaning that the diffusion of photo-active species affects the cells’ ability to function. In this cell we used very dilute solution of dye as photosensitizer, reductant, as electron donor / acceptor from dye and surfactant for assisting solubility and stability of system, in an alkaline medium. First of all, Becquerel 1 discovered the generation of current and voltage under the impact of solar radiation on iron electrode, which make the basis of solar cell. Further on, Rideal and Williams 2 worked on kinetic study of bleaching of thionine dye in presence of ferrous salt. Photogalvanic term was first used by Rabinowitch3,4 and systematically analyzed. Thionine based work was processed for photophysical charge 5. Tamilarasan and Natarajan6 studied photogalvanic cell with two platinum electrode, one in dark and another in illuminated demonstrate a cyclic process for generation of photovoltage in PG cell. Dye-sensitized solar cells (DSSCs) were prepared using various food dyes. Food dyes are economically superior to organ metallic dyes since they are nontoxic and inexpensive7. The mixed surfactant based sensitized dye interaction results were studied for electrical output8. The mixed surfactant base results are reported for better electrical output by Lal and Gangotri9. Later on Meena at al., observed the photochemical degradation of dye 10, Yadav et al. studied photo reduction of methylene blue and Safranine O in EDTA11. Rathore et al. 12,13 the short-lived processes in PG cells for solar power generation and storage. Rathore and Lal studied photogalvanics for comparative better electrical output 14. One of the greatest challenges in the last decade has been discovering new energy sources with minimum toxicity 15. The relevant literature survey was done about photogalvanic cells have been used in photo induced generation of power and storage 16. Lal and Gangotri . studied photogalvanics of mixed surfactant system17. The innovative results about methylene blue with mixed surfactant were reported in account of solar energy field 18. These results are published in environment science and pollution research journals. On the basis of relevant survey, the comparative study of synthetic food dyes with reductant surfactant system in photogalvanic cell for photo induced power generation and storage was taken for better results.
Material and Method
Materials
1. |
Photosensitizer – Tatrazine, Sunset yellow |
6. |
A – Microammeter |
2. |
Reductant – EDTA (Ethylenediamine tetraacetic acid) |
7. |
F – Water Filter |
3. |
Surfactant – DSS (Dioctyl sodium sulphosuccinate) |
8. |
S – Light Source |
4. |
SCE – Saturated calomel electrode |
9. |
V – pH Meter |
5. |
Pt electrode – Platinum electrode |
10. |
PG – Photogalvanic |
Method
The photogalvanic cell was fabricated using glass material, the shape of cell like alphabet H, this H-shaped cell covered by some adhesive black material which do not allow photo bleaching of dye solution along with reductant surfactant solution of various concentration up to 25 ml in alkaline medium of high pH range. There is a transparent window was present in one compartment of H cell. Place the SCE in the dark part in PG cell setup and the pt- electrode as the anode in the other bright compartment. Electrode terminals are connected to carbon pot and (resistor) switches. Photopotential and photocurrent were determined with a pH meter and micro ammeter photogalvanic cells. An electrical bulb is utilized at low-power consumption and water filter was used to block IR – rays as it cause thermal effects which results in low performance of PG cell. Stock solution of M/100 concentration was prepared in double distilled water. Isc of a given photogalvanic cell measured using a micrometer by keeping circuit closed and Voc measured using a digital pH-meter keeping the circuit open of both systems. 470k carbon pots were applied in the micro ammeter circuit, through by outer electric load applied to measure the peak value of photopotential and photocurrent. Power is measured as the power concept, the current at the Pp and the potential at Pp are called ipp and Vpp. Cell performance is measured as t½ of the cell. The time is observed in the dark when the cell’s performance reaches half its value. This value indicates the storage of PG cell and this cell can work in the dark.
![]() |
Figure 1: Experimental Pg Cell Setup |
Result And Discussion
Optimum concentration of anionic Surfactant DSS for optimal performance of photogalvanic cell
Surfactant play an important role in improving performance of photogalvanic cell, they are not essential for photogalvanic cell. Photogeneration of Voltage and current also possible in their absence but their presence show encouraging impact on conversion efficiency, Surfactant are employed for solubilizing dye and also provide stability to the system. It was found that optimal concentration of DSS at which performance of the cell was most favorable at (1.76 x 10-3 M). Below this concentration value the current of the PG cell was low as less number of DSS molecules present for assisting in solubility of dye molecule. Current potential parameters of the cell had shown decreasing trend after reaching optimal value as outsized molecule of surfactant obstruct the motion of dye molecule towards the electrode. The outcomes are reported in Table no.1.
Table 1: Optimum Concentration of Anionic Surfactant (DSS) in Both the System:
TATRAZINE+EDTA+DSS SYSTEM |
SY+EDTA+DSS SYSTEM |
||||||
Concentration of DSS × 10-3 M |
Pp (mV) |
Pc (µA) |
POWER (µW) |
Concentration of DSS × 10-3 M |
Pp (mV) |
Pc (µA) |
POWER (µW) |
1.60 |
728.0 |
168.0 |
122.30 |
1.60 |
733.0 |
307.0 |
225.03 |
1.68 |
813.0 |
197.0 |
160.16 |
1.64 |
813.0 |
352.0 |
286.18 |
1.76 |
879.0 |
220.0 |
193.38 |
1.68 |
864.0 |
390.0 |
336.96 |
1.84 |
808.0 |
198.0 |
159.98 |
1.72 |
800.0 |
348.0 |
278.40 |
1.92 |
732.0 |
172.0 |
125.90 |
1.76 |
722.0 |
301.0 |
217.32 |
# SY: Sunset Yellow, EDTA: Ethylenediamine Tetraacetic Acid, DSS: Dioctyl Sodium Sulphosuccinate.
Impact of variation in Dyes (Tatrazine and sunset yellow)
The Tatrazine and SY were highly soluble in water and transparent solutions of both dyes were formed in water, variation in the concentration of food dye shows impact on the electrical parameters of cell. Photogalvanic cell showed optimum results at a very dilute concentration of the dye (2.0 x 10-5 M). At higher concentration aggregations of dye molecules causes low electrical performance in photogalvanic cell. Table 2 displays how variations in the concentration of photosensitizer (Tatrazine and SY) affect electrical output in DSS-EDTA systems.
Table 2: Optimum Concentration of Dye (Tatrazine and Sunset Yellow) in Both the System
TATRAZINE+EDTA+DSS SYSTEM |
SY+EDTA+DSS SYSTEM |
||||||
Dye concentration x 10-5 M |
Pp (mV) |
Pc (µA) |
POWER (µW) |
Dye concentration x 10-5 M |
Pp (mV) |
Pc (µA) |
POWER (µW) |
1.20 |
712.0 |
172.0 |
122.46 |
1.2 |
722.0 |
330.0 |
238.26 |
1.60 |
805.0 |
195.0 |
156.98 |
1.4 |
801.0 |
363.0 |
290.76 |
2.00 |
879.0 |
220.0 |
193.38 |
1.6 |
864.0 |
390.0 |
336.96 |
2.40 |
813.0 |
194.0 |
157.72 |
1.8 |
788.0 |
352.0 |
277.38 |
1.80 |
715.0 |
168.0 |
120.12 |
2.0 |
712.0 |
310.0 |
220.72 |
# SY: Sunset Yellow, EDTA: Ethylenediamine Tetraacetic Acid, DSS: Dioctyl Sodium Sulphosuccinate.
Impact of variation in (EDTA) electrical output of system
The PG cells having tartrazine + EDTA + DSS system and SY + EDTA + DSS increased with decreasing agent (reductant) concentration and reached the maximum value under agreement, indicating the negative of the current small scale. The presence of reducing agent molecules helps the dye molecules donate electrons and increases its concentration after reaching the best concentration, when there is no trend of decreasing current in the photogalvanic cell, it will find that further concentration of electron donating molecules is reliable. The reverse rate of flow from the electron transfer molecule to the electron donor group from the dye molecule will also block the path of the photosensitizer molecule to the electrode.
Impact of Diffusion length on the electrical output
Diffusion length is the distance between electrodes. Photogalvanic cells are diffusion controlled and the operation of these cells depends on the diffusion of ions. As the diffusion length increase, the volume of the electrolytic solution on the electrodes increases and the conductivity of the solution also increases, which increases the current in the photogalvanic cell. Larger solutions are more common. During the optimum diffusion length is reached at maximum and the increase in the number of excited photosensitizing species reaching the platinum electrode and during its short lifetime result in a decrease in ignition. Because the excited dye molecules will not be able to reach the platinum electrode in time. As the diffusion length of the electrode change, current parameters such as imax and isc are examined and it is seen that the diffusion length increases, the maximum photocurrent (imax).The current production rate (mAmin-1) increase, but there is a micro and invisible decrease in the short circuit current (isc) changes in diffusion length adversely affect downfall. Table 3 shows the effect of diffusion length in the photosensitizer EDTA – DSS system on the available parameters.
Table 3: Impact of Diffusion Length on Current Parameters
TATRAZINE+EDTA+DSS SYSTEM |
SY+EDTA+DSS SYSTEM |
||||||
DL(mm)
|
Imax (µA) |
Ieq(µA) |
Rate of initial generation of photocurrent (µA mn-1) |
DL (mm)
|
Imax (µA) |
Ieq (µA) |
Rate of initial generation of photocurrent (µA min-1) |
50 |
272.0 |
228.0 |
6.80 |
50 |
448.0 |
400.0 |
14.933 |
55 |
276.0 |
224.0 |
6.90 |
55 |
454.0 |
394.0 |
15.133 |
60 |
280.0 |
220.0 |
7.00 |
60 |
460.0 |
390.0 |
15.333 |
65 |
284.0 |
216.0 |
7.10 |
65 |
466.0 |
386.0 |
15.533 |
70 |
288.0 |
214.0 |
7.20 |
70 |
472.0 |
382.0 |
15.733 |
# SY: Sunset Yellow, EDTA: Ethylenediamine Tetraacetic Acid, DSS: Dioctyl Sodium Sulphosuccinate.
pH Variation effect
There are many studies showing the significant effects of alkalinity of solution on photovoltaic cell performances, and many studies have shown that the current of photovoltaic cells in the alkaline range increases with increasing ionization. We prepare a 1N sodium hydroxide solution and the pH of the solution needs to be raised or lowered by changing the volume of the mixture consisting of 25 ml of dye, surfactant and reducing agent. Photogalvanic cells containing tartrazine and sunset yellow + EDTA + DSS systems showed improvement in electrical properties with increasing pH, reaching maximum pH (pH = 12.60), and the voltage output of the system decreased with increasing pH. Table 4 shows the results of pH changes in both systems.
Table 4: Ph Variation Effects
TATRAZINE+EDTA+DSS SYSTEM |
SY+EDTA+DSS SYSTEM |
||||||
PH |
Pp (mV) |
Pc (µA) |
POWER (µW) |
PH |
Pp (mV) |
Pc (µA) |
POWER (µW) |
12.52 |
702.0 |
151.0 |
106.0 |
11.39 |
732.0 |
277.0 |
202.76 |
12.56 |
797.0 |
185.0 |
147.45 |
11.60 |
798.0 |
345.0 |
275.31 |
12.60 |
879.0 |
220.0 |
193.38 |
11.78 |
864.0 |
390.0 |
336.96 |
12.63 |
802.0 |
187.0 |
149.97 |
11.96 |
786.0 |
333.0 |
261.74 |
12.66 |
705.0 |
155.0 |
109.28 |
12.15 |
721.0 |
276.0 |
199.00 |
# SY: Sunset Yellow, EDTA: Ethylenediamine Tetraacetic Acid, DSS: Dioctyl Sodium Sulphosuccinate.
Influence of changing of electrode area
The electrode area of the platinum electrode has a major impact on the cell’s electrical performance. The (imax) and (ieq) of a photogalvanic cell with Tatrazine and sunset yellow – EDTA – DSS system were measured by adjusting the platinum electrode’s area. Optimum results were obtained at 1×1 cm2 electrode area.
Table 5: Influence of Changing Electrode Area (EA) on Parametars of Photogalvanic Cell
TATRAZINE+EDTA+DSS SYSTEM |
SY+EDTA+DSS SYSTEM |
||||
Electrode Area (CM2) |
imax (µA) |
ieq (µA) |
Electrode Area (CM2) |
imax (µA) |
ieq (µA) |
0.70 |
270.0 |
228.0 |
0.70 |
452.0 |
398.0 |
0.85 |
275.0 |
224.0 |
0.85 |
456.0 |
394.0 |
1.00 |
280.0 |
220.0 |
1.00 |
460.0 |
390.0 |
1.15 |
285.0 |
216.0 |
1.15 |
464.0 |
386.0 |
1.30 |
290.0 |
214.0 |
1.30 |
484.0 |
382.0 |
# SY: Sunset Yellow, EDTA: Ethylenediamine Tetraacetic Acid, DSS: Dioctyl Sodium Sulphosuccinate.
(i-V) features of the photogalvanic cell
The current-voltage (i-V) characteristics of PG cell in the Tatrazine and SY + EDTA + DSS Systems, respectively are displayed in fig. 2A and 2B.
![]() |
Figure 2: A and B (i-V) Current – Voltage characteristics of the both cell |
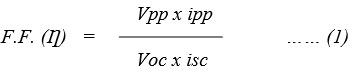
Where
Vpp = Value of potential at power point in dye EDTA-DSS system.
ipp = Value of current at power point in dye EDTA-DSS system.
Voc = Represent open circuit voltage in dye EDTA-DSS system.
isc = Represent short circuit current in dye EDTA-DSS system.
Cell performance and conversion efficiency
Reported cell can take energy from the cell in the dark up to t1/2time, which was measured 100 minutes and 140 minutes. The utilizing of PG cell was detected in the dark using an external load by stabilizing the system at its power point current, as a constant value of the potential reached by the system. Power was noted in terms of t1/2, i.e. (power) to drop to half of that at the power point. The CE was determined to be 0.6163% and 1.2186% using below equation (2):

The Vpp, ipp, and A – area are Tatrazine and sunset yellow – EDTA – DSS system; 493 mV, 130µA, 1.0 ⅹ 1.0 cm2 and 551.0 mV, 230 µA, 1.0 ⅹ 1.0 cm2 respectively.
Mechanism
Photogeneration of current by falling solar radiation in PG cell system was drafted through a mechanism.
Excitation Reactions
First of all dye absorbs sun radiation reaches up to excited state (singlet state) this state loses energy converts into more stable excited species triplet state, this form of dye gain the e– from the reducing agent and is transfer to a reduced to photosensitizer form of the dye, which releases an e– flow on platinum electrode is converted to an uncharged dye molecule, an electrons by the outer circuit reaches the calomel electrode of the dark chamber.
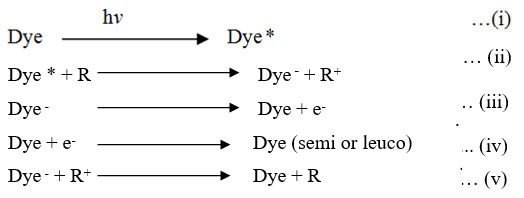
Given Dye, Dye–, R and R+ is the dye (Tatrazine and SY), its leuco form, reducing agent (EDTA) and oxidized form.
Conclusion
Based on the obtained, it is probably increase performance of the photogalvanic cell by changing different concentrations of dye, reducing agent, and surfactant. Largely in terms of conversion and storage the Sunset yellow + DSS + EDTA system in the photogalvanic cell is more efficient to storage solar energy than the Tatrazine + DSS + EDTA system in our research work. Therefore, a better choice of dye and surfactant affects the overall efficiency, and we can state that efficient photogalvanic cells can be developed in systems with a dye with a high absorption spectrum. We used low-cost food dyes, which are no longer life-threatening, to produce a low-cost photogalvanic cell. The surfactant plays an important role in improving the cell performance by suppressing the thermal back-transfer of electrons, also ensuring the smooth conduction of the electron into the dye molecule and subsequently optimizing the concentration of the platinum electrode in the solutions used. It is suggested that we should go for cheap and biodegradable chemicals at low solution concentrations along with their higher stability in systems to investigate the economical way to sustainability and environmental friendliness of these cells. Conclusively photogalvanic cell offer future prospective for low cost more sustainable solar cell employing suitable dye-reductant-surfactant combinations.
Acknowledgment
The authors thankful to the HOD, Department of Chemistry, J.N.V.U., Jodhpur, Rajasthan, INDIA for the scientific analysis and providing all necessary equipment.
Conflict of Interest
There is no conflict of interest.
References
- Becquerel, E., Comptes Rendus Paris, 1839, 9, 561-567.
- Rideal, E.K.; Williams, E.G., J. Chem. Soc. Trans., 1925, 127, 258-269.
CrossRef - Rabinowitch, E., J. Phy. Chem., 1940, 8, 551-559.
CrossRef - Rabinowitch, E., J.Phy. Chem., 1940, 8, 560-566.
CrossRef - Singhal, G.S.; Rabinowitch, E., J. Chem. Phy., 1970, 53, 4109-4110.
CrossRef - Tamilarasan, R., Natarajan, P., Nature, 1970, 292, 224–225.
CrossRef - Hosseinnezhad, M.; Rouhani, S., Opto. Electronics Review, 2016, 24, no.1, 34-39.
CrossRef - Mohan, L.; Gangotri, KM., J. Solar Energy Res., 2022, 7(3), 1095-1103.
- Lal, M.; Gangotri, K.M., Res. J. Rec. Sci., 2013, 2(12), 19-27.
- Chandra, M.; Singh, A.; Meena, R.C., Int. J.Phy. Sci., 2012, 7, 5642-5648.
- Yadav, S.; Lal, C., Energy Sources Part A., 2010, 32, 1028-1039.
CrossRef - Rathore, J.; Arya R. K.; Sharma P.; Lal M., Res. J. Chem. Environ., 2022, 26 (6),24-29.
CrossRef - Rathore, J.; Arya R. K.; Sharma P.; Lal M., Indian journal of science and technology,2022, 15(23), 1159–1165.
CrossRef - Rathore, J.; Lal M.,Res. J. Chem. Environ, 2018, 22 (6),53-57.
CrossRef - Hosseinnezhad, M.; Moradian, S.; Gharanjig, K., Prog. Colour Colorants Coat, 2013, 6, 109–117.
- Sharma, P.; Rathore, J., Orient. J. Chem., 2023,39(4), 1059-1063.
CrossRef - Lal, M.; Gangotri, KM., Int. J. Energy Res., 2022, 46(14), 19538-19547.
CrossRef - Lal, M.; Gangotri, KM., Environ Sci Pollut Res., 2023, 30(44), 98805-98813.
CrossRef
Abbreviation
1. |
Voc = Open circuit voltage |
10. |
t1/2 = Performance of cell |
2. |
isc = Short circuit current |
11. |
C.A.= Conversion efficiency |
3. |
Vpp = Photo potential at power point |
12. |
ml = Milliliter |
4. |
ipp = Photocurrent at power point |
13. |
mV = Millivolt |
5. |
Ppp = Power at power point |
14. |
mA = Microampere |
6. |
imax = Maximum photocurrent |
15. |
mW = Microwatt |
7. |
ieq = Photocurrent at equilibrium |
16. |
M = Morality |
8. |
DL = Diffusion length |
17. |
SY = Sunset Yellow |
9. |
ff = fill factor |
18. |
PG = Photogalvanic |
This work is licensed under a Creative Commons Attribution 4.0 International License.