Kinetics of Thiazole Formation Using α-Halo Ketones and Thioamides
Prajakta Shamrao Sadavarte, Bhaskar Hari Zaware*
and Sushama Jayawant Takate
Department of Chemistry, New Arts, Commerce and Science College, Ahmednagar, Maharashtra, India.
Corresponding Author E-mail: bhaskarzaware@gmail.com
DOI : http://dx.doi.org/10.13005/ojc/380427
Article Received on : 27 Jun 2022
Article Accepted on : 13 Aug 2022
Article Published : 24 Aug 2022
Reviewed by: Dr. Vijay Bhamare
Second Review by: Dr. Daniel
Final Approval by: Dr. Charanjit Kaur
The current approach involves the kinetic investigation of Thiobenzamide (TB) and cyclohexanone thiosemicarbazone (CTSC) in solution with 3-chloroacetylacetone(3-CAA). The study revealed a second-order rate constant with respect to both reactants, thioamide and an α-halo carbonyl compound. The energy of activation (Ea) was found to be Ea 48.8176 kJ/mol/K for thiobenzamide and 33.08692157 kJ/mol/K for Cyclohexanone thiosemicarbazone. The salt effect study showed the formation of ionic species during the reaction. The reactions were studied with respect to changes in dielectric constant using solvent systems like water-ethanol and water-isopropanol.
KEYWORDS:Cyclisation; Dielectric Constant; Kinetics; Mechanism; Thermodynamic parameter
Download this article as:
Copy the following to cite this article: Sadavarte P. S, Zaware B. H, Takate S. J. Kinetics of Thiazole Formation Using α-Halo Ketones and Thioamides. Orient J Chem 2022;38(4). |
Copy the following to cite this URL: Sadavarte P. S, Zaware B. H, Takate S. J. Kinetics of Thiazole Formation Using α-Halo Ketones and Thioamides. Orient J Chem 2022;38(4). Available from: https://bit.ly/3AHGNvr |
Introduction
Hantzsch thiazole cyclization is the crucial synthetic route for thiazole-containing compounds which have numerous applications. Thiazoles are found in a variety of natural products, including carboxylase vitamin B1, thiamine pyrophosphate (TPP), and penicillin1, many of the compounds which are in current clinical use for bacterial infection2, allergies3, HIV infections4, hypertension5, as hypnotic agents6, schizophrenia7 and pain8 and inhibition of bacterial DNA gyrase B9 possess thiazole as part of the structure. Thiazole anchored molecules exhibit antifungal10, anti-inflammatory11-12, analgesic13, anticonvulsant 14, anti-cancer 15-16 and antimicrobial 17-19 properties also.
The current work reports on pH metric measurements of the cyclisation of thioamides and 3-choroactylacetone in a water-ethanol system to observe the concentration of H+ ion in the solution phase.
Available literature shows that so far very less work has been carried out on the kinetics of thiazole formation. We previously used 3-chloroacetylacetone and substituted thioureas to investigate thiazole formation20. The reaction between 3-chloroacetylacetone and thioamides is still to be reported. As it is unexplored for its kinetic aspect, it must be studied for the same. In 1884, Van’t Hoff 21 proposed a method for calculating rates based on the slope of a concentration versus a time plot.

The cage model was utilized by Robinowitch22 to improve the collision theory for second-order processes in solution. According to the transition state theory23, which gives a straightforward way of putting together reaction rates and how the process occurs. According to Erying and Laidler24, the energy barrier is required for product formation, which provides the activation energy through the development of the activated complex. The energy of activation Ea and preexponential factor-A are computed using the Arrhenius equation in the solution phase reaction as the temperature is varied25.
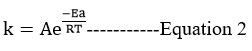
It was estimated the activation energy, preexponential factor, activation entropy, and free energy.

For bimolecular reaction,
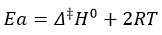
The solvent binding is shown to restrict the free movement of molecules by negative entropy26. When entropy is lost, an activated complex is generated.
According to the salt effect, when the interacting species are oppositely charged, the rate drops as the ionic strength increases.

Debye and Huckel27 and Bronsted28

Logk and √I (mol dm-3) A straight line with a slope of 1.02zAzB is seen in the graph. The rate-determining phase of the reaction is between oppositely charged species, which has a negative slope29,32 and indicates that oppositely charged species are present in the reaction. The data influence of the dielectric constant is explored by Akerlof30, and the data is used in this study. The dielectric constants for the composition of water-ethanol and water-isopropanol systems were obtained using the supplied standard graph.
Using the double sphere model, Scatchard’s31 equation is employed for the mixed solvent with variable dielectric constant, according to electrostatic theory. The logarithm of the rate constant plotted against the reciprocal of the dielectric constant yields a linear curve24. It has been observed that there is some variation from linearity at low values of dielectric constants.

![]() |
Scheme 1: Reaction between thioamide with 3-chloroacetyl acetone. |
Materials and Methods
A digital pH meter was used to determine the change in pH with the progress of the reaction. (EQUIPTRAONICS, EQ-614A). All of the reagents employed were analytical reagents. Glass distillated water and pure alcohol were used to perform the experiment.
Experimental
Four different parts were carried out for the kinetic study of the reaction.
Effect of concentration
To evaluate the order of reaction in the case of -halo ketone, the reaction was carried out with various concentrations of α-halo ketone while maintaining the concentration of thioamide constant (0.05N) at 300K. By plotting a graph of H+ concentration versus time in minutes, the dc/dt values were calculated. By drawing a tangent at a specific time, the values of dc/dt were computed, as well as the sequence of reactions for each reactant. The slope of the log dc/dt graph versus and found to be nearly one. With respect to 3-chloroacetyl acetone, this observation shows that the reaction is first order.
Effect of variation of temperature
The reaction between two different substituted thioamides and 3-chloroacetyl acetone was studied kinetically using equal concentrations at 300K, 303K, 308K, 3013K, 318K, and 323K. The activation energy (Ea)reactions were determined by plotting 1/T vs log k.
Effect of salt addition
The reaction between two distinct substituted thioamides and 3-chloroacetyl acetone was studied kinetically using equal amounts of sodium chloride and lithium chloride at 300K. The slope of the plot of õ versus log k is negative.
Effect of solvent
The reaction between two distinct substituted thioamides and 3-chloroacetyl acetone was studied kinetically at 300K utilizing the Water-Ethanol and Water-Isopropyl alcohol systems at equal concentrations. By using the double sphere mechanism, plotting 1/T vs log k gives a negative value, which indicates the dAB distance between two ions.
Table 1: Order of the reaction with respect to one reactant.
Reactants |
Order |
Reactants |
Order |
TB change |
1.1471 |
CTSC change |
1.10872 |
3-CAA change |
0.5339 |
3-CAA change |
1.17052 |
Table 2: Temperature effect and thermodynamic parameters: Thioamide reacts with 3-chloroacetylacetone.
Temperature (0C)
|
Ea kJ/mol/K |
∆H‡ kJ/mol/K
|
∆S‡ kJ/mol |
∆G‡ kJ/mol |
Thiobenzamide |
48.8176 |
43.8292 |
-0.1282 |
82.3009 |
Cyclohexanone Thiosemicarbazone |
33.0869 |
28.0985 |
-0.1635 |
77.1645 |
Table 3: Rate constants for salt effect (dm3mol-1s-1).
Concentration Salt effect
|
0.1N |
0.2N |
0.3N |
0.4N |
TB and 3CAA (NaCl) |
5.34X10-3 |
5.09X10-3 |
4.86X10-3 |
4.12X10-3 |
TB and 3CAA (LiCl) |
4.61X10-3 |
3.76X10-3 |
3.53X10-3 |
3.37X10-3 |
CTSC and 3CAA (NaCl) |
2.85 X10-2 |
2.12 X10-2 |
2.02 X10-2 |
1.82 X10-2 |
CTSC and 3CAA (LiCl) |
1.90 X10-2 |
1.74 X10-2 |
1.58 X10-2 |
1.39 X10-2 |
Table 4: Rate constant in the water-ethanol system (dm3mol-1s-1).
TB and 3-CAA reaction |
Rate (Water-Ethanol system) |
Rate (Water-Isopropanol system) |
85-15 |
1.73 X10-3 |
3.31 X10-3 |
75-25 |
1.87 X10-3 |
3.46 X10-3 |
65-35 |
2.27 X10-3 |
3.93 X10-3 |
55-45 |
2.79 X10-3 |
4.33 X10-3 |
Table 5: Rate constant in the water-Isopropanol system (dm3mol-1s-1).
CTSC and 3-CAA reaction |
Rate (Water-Ethanol system) |
Rate (Water-Isopropanol system) |
85-15 |
1.55 X10-2 |
6.38 X10-4 |
75-25 |
2.06 X10-2 |
7.60 X10-4 |
65-35 |
2.19 X10-2 |
9.88 X10-4 |
55-45 |
2.34 X10-2 |
1.37 X10-3 |
General mechanism and rate expression are proposed
![]() |
Figure 1: Rate constant for the second-order reaction between α-halo ketone with thioamides. |
Result and Discussions
Using Van’t Hoff’s differential method, the plot of log (dc / dt) against log 3-chloroacetylacetone and log thioamide is a straight line with a slope of nearly one with respect to both, and the stoichiometric study suggested that one mole of thioamide reacts with one mole of 3-chloroacetylacetone. (Table I, Figure 3,4). The reaction takes place in a two-step sequence. As the reaction is performed at moderate temperatures and no free radical initiators are used, there is no possibility of free radical formation. The second-order rate constants were investigated at various temperatures. Other thermodynamic parameters were derived by studying the reaction at various temperatures. The energy of activation (Ea) was established by producing a graph of log k versus 1/T. (Table II, Figure 5,6). The reaction’s entropy of activation (S*) is negative, indicating that the transition state is inflexible. When (H*) is negative, the reaction is exothermic. The reaction is exothermic when (H*) is negative. The activation entropy (S*) is negative, indicating that less stable noncyclic reactants transform into stable cyclic products. The activation entropy has a negative value, indicating stiffness in the transition state. The reaction could happen between ions with the same charge. The nucleophile and electrophile moieties are involved in the reaction, as seen by the negative slope of 1/T versus log k. (Table III, Figure 7,8). The dAB is obtained by plotting the 1/T vs log k values (distance between two ions by double sphere mechanism). The reaction is faster in the water-isopropyl alcohol system than in the water-ethanol system. (Table IV, V Figure9,10). According to the kinetic theory of collision, if the ions are of opposite sign, the frequency will increase due to the attraction force.
![]() |
Figure 2: Order of the reaction between Thiobenzamide and 3-Chlolroacetylacetone. |
![]() |
Figure 3: Order of the reaction between 3-Chloroacetylacetone and Cyclohexanone thiosemicarbazone |
![]() |
Figure 4: Temperature change of the reaction between Thiobenzamide and 3-Chlolroacetylacetone |
![]() |
Figure 5: Temperature change of the reaction between 3-Chloroacetylacetone and Cyclohexanone thiosemicarbazone. |
![]() |
Figure 6: Salt effect on Thiobenzamide and 3-Chlolroacetylacetone. |
![]() |
Figure 7: Salt effect on 3-Chloroacetylacetone and Cyclohexanone thiosemicarbazone. |
![]() |
Figure 8: Solvent effect on Thiobenzamide and 3-Chlolroacetylacetone. |
![]() |
Figure 9: Solvent effect on 3-Chloroacetylacetone and Cyclohexanone thiosemicarbazone |
Conclusion
Using Van’t Hoff’s differential approach, the reaction between 3-chloroacetylacetone and thioamides is kinetically examined to establish second-order rate constants.
The second-order rate of the reaction is also shown by the rate law.
Rate of the reaction is directly proportional to temperature. At lower temperature reaction rate will be slowed down.
Negative entropies cause the open-chain compounds to form a cyclic molecule. It is possible to have nucleophilic addition and addition–elimination processes.
There is a reaction between oppositely charged moieties such as nucleophile and electrophile.
As the dielectric constant rises, the rate rises as well. In comparison to the ethanol-water system, the isopropyl alcohol-water system has a high-rate constant.
Acknowledgement
They expressed gratitude to the A.J.M.V.P. Samaj’s, Ahmednagar (M.S.) authorities for their assistance and research facilities.
Conflict of Interest
There is no conflict of interest.
Funding Sources
There is no funding source.
References
- Karam, N. H.; Tomma, J. H.; Al-Dujaili, A. H., Chem. Mater. Res. 2013, 3(9), 162–171.
- Sharma, R. N.; Xavier, F. P.; Vasu, K. K.; Chaturvedi, S. C.; Pancholi, S. S., J. Enzyme Inhib. Med. Chem. 2009, 24, 890–897.
CrossRef - Haragave, K. D.; Hess, F. K.; Oliver, J. T., J. Med. Chem. 1983, 26(8), 1158–1163.
CrossRef - Bell, F. W.; Cantrell, A. S.; Hoegberg, M.; Jaskunas, S. R.; Johansson, N. G.; Jordan, C. L.; Kinnick, M. D.; Lind, P.; Morin, J. M., J. Med. Chem. 1995, 38, 4929–4936.
CrossRef - Patt, W. C.; Hamilton, H. W.; Taylor, M. D.; Ryan, M. J.; Taylor, D. G.; Connolly, C. J. C.; Doherty, A. M.; Klutchko, S. R.; Sircar, I., J. Med. Chem. 1992, 35, 2562–2572.
CrossRef - Ergenç, N.; Çapan, G.; Günay, N.S.; Özkirimli, S.; Güngör, M.; Özbey, S.; Kendi, E. Arch. Pharm. Pharm. Med. Chem..1999, 332, 343–347 .
CrossRef - Carter, J. S.; Kramer, S.; Talley, J. J.; Penning, T.; Collins, P.; Graneto, M. J.; Seibert, K.; Koboldt, C. M.; Masferrer, J.; Zweifel, B., Bioorg. Med. Chem. Lett. 1999, 9, 1171–1174.
CrossRef - Rudolph, J.; Theis, H.; Hanke, R.; Endermann, R.; Johannsen, L.; Geschke, F. U., J. Med. Chem. 2001, 44, 619–626.
CrossRef - Lino, C. I.; Gonçalves de Souza, I.; Borelli, B. M.; Silvério Matos, T. T.; Santos Teixeira, I. N.; Ramos, J. P.; Maria de Souza Fagundes, E.; de Oliveira Fernandes, P.; Maltarollo, V. G.; Johann, S.; de Oliveira, R. B., Eur. J. Med. Chem. 2018, 151, 248–260.
CrossRef - Sinha, S.; Doble, M.; Manju, S. L., Eur. J. Med. Chem. 2018, 158, 34–50.
CrossRef - Kamble, R. D.; Meshram, R. J.; Hese, S. V.; More, R. A.; Kamble, S. S.; Gacche, R. N.; Dawane, B. S., Comput. Biol. Chem. 2016, 61, 86–96.
CrossRef - Pember, S. O., Mejia, G. L., Price, T. J. & Pasteris, R. J., Bioorg. Med. Chem. Lett. 2016, 26, 2965–2973.
CrossRef - Amin, K. M.; Rahman, A. D. E.; Al-Eryani, Y. A., Bioorg. Med. Chem. 2008, 16, 5377–3588.
CrossRef - Wang, Y.; Wu, C.; Zhang, Q.; Shan, Y.; Gu, W.; Wang, S., Bioorg. Chem. 2019, 84, 468–477.
CrossRef - da Santana, T. I.; Barbosa, M. O.; Gomes, P. A. T. M.; da Cruz, A. C. N.; da Silva, T. G.; Leite, A. C. L., Eur. J. Med. Chem. 2018, 144, 874–886.
CrossRef - Reddy, G. M.; Garcia, J. R.; Reddy, V. H.; de Andrade, A. M.; Camilo, A.; Pontes R., Renan A.; de Lazaro, S. R., Eur. J. Med. Chem. 2016, 123, 508–513.
CrossRef - Cushman, M.S.; Seleem, M.; Mayhoub, A. S., United States Patent No.: US 9, 801, 861 B2, 2017.
CrossRef - Leoni, A.; Locatelli, A.; Morigi, R.; Rambaldi, M., Expert Opin. Ther. Patents 2014, 24, 201–216.
CrossRef - Zaware, B. H.; Mane, R. A.; Kuchekar, S. R., J. Chem. Pharma. Res. 2009,1(1) 276.
- Van’t Hoff. J. H., Multer compony, Amsterdam, 1984.
- Rabinovitch, E., Trans. Faraday Soc. 1937, 33,1225.
CrossRef - Laidler, K. J.; King, M. C., J. Phys. Chem., 1983, 87, 2657.
CrossRef - Laidler, K. J.; Eyring, H., Ann. N. Y. Acd. of sciences., 1940, 39,303.
CrossRef - Arrhenius, S., J. Phys. Chem., 1889, 4, 226.
CrossRef - Glassstone, S., J. Chem. Soc. 1936, 723, Laidler, K. J. Chem.Kinetic., 195,3rd ed.,2009.
- Debye, P. J. W. and Huckel, E., Phys. Z., 1923, 24, 185, 305.
- Bronsted, J. N.; La Mer, V. K., J. Am. Chem. Soc. 1924,46, 555.
CrossRef - Jonnalagadda, S. B.; Gollapalli, N. R., J. Chem. Edu., 2000, 77,4.
CrossRef - Akerlof, G., J. Am. Chem. Soc., 1932, 54, 4125.
CrossRef - Scatchard, G., Chem. Rev. 1932, 10,229.
CrossRef - Stokes, R. H.; Robinson, R. A., J. Am. Chem. Soc., 1948, 70, 1870.
CrossRef
This work is licensed under a Creative Commons Attribution 4.0 International License.