Synthesis and Characterization of ZnO@SiO2 Nanocomposite using Gum Arabic and its Larvicidal Activity on Malaria Vectors
Zaccheus Shehu1*, Ezra Abba2
, Zainab Adamu Abubakar3
, Rifkatu Kambel Dogara4
and Nsor Charles Ayuk2
1Department of Chemistry, College of Natural Science, Makerere University, Kampala, Uganda.
2Department of Zoology, Faculty of Science, Gombe State University, Gombe PMB 127, Nigeria.
3Department of Biological Sciences, Faculty of Science, Gombe State University, Gombe PMB 127, Nigeria.
4Department of Chemistry, Faculty of Science, Gombe State University, Gombe PMB 127, Nigeria.
Corresponding Author E-mail: zaccheusshehu@gmail.com
DOI : http://dx.doi.org/10.13005/ojc/380313
Article Received on : 10 Mar 2022
Article Accepted on :
Article Published : 17 May 2022
Reviewed by: Dr. Ali N Sabbar
Second Review by: Dr. M. Deore
Final Approval by: Dr. Pounraj Thanasekaran
Green synthesis of ZnO@SiO2 nanocomposite was performed using Gum Arabic. The green synthesized nanocomposite was characterized using Fourier Transform Infrared Spectrophotometer (FTIR), The Scanning Electron Microscopy (SEM), UV-Visible spectrophotometer, Dynamic Light Scattering (DLS), and X-Ray Fluorescence (XRF). The toxicity study was conducted for 24 hours on 1st to 4th larval instars of malaria vectors at various concentrations (10, 20 and 25 mg/L). The LC50 and LC90 for the 1st - 4th larval instars were found to be in the range of 9.11 - 18.288 mg/L and 157.254 - 126.132 mg/L respectively. A strong positive correlation between concentrations of the nanocomposite and mortality of larval instars (0.945 - 0.997). These values indicate that the mortality rates increased with an increase in concentrations. ZnO@SiO2 nanocomposite is a potential nano-larvicide for malaria vector control in tropical countries with high malaria incidence.
KEYWORDS:Green synthesis; Gum Arabic; Malaria Vectors; Nanocomposite; ZnO@SiO2
Download this article as:
Copy the following to cite this article: Shehu Z, Abba E, Abubakar Z. A, Dogara R. K, Ayuk N. C. Synthesis and Characterization of ZnO@SiO2 Nanocomposite using Gum Arabic and its Larvicidal Activity on Malaria Vectors. Orient J Chem 2022;38(3). |
Copy the following to cite this URL: Shehu Z, Abba E, Abubakar Z. A, Dogara R. K, Ayuk N. C. Synthesis and Characterization of ZnO@SiO2 Nanocomposite using Gum Arabic and its Larvicidal Activity on Malaria Vectors. Orient J Chem 2022;38(3). Available from: https://bit.ly/3Ml2tkp |
Introduction
Malaria is one of the common life-threatening diseases mostly found in the tropics. Over 100 hundred countries were reported to be at risk of transmission of this disease. It is caused by protozoan parasites of the genus Plasmodium. Most human malaria is caused by four different species of the Plasmodium parasite: Plasmodium falciparum, P. malariae, P. ovale and the P. vivax 1. The world malaria report indicated that 228 million malaria cases were reported worldwide, out of which 405,000 died. Africa accounted for the majority of the malaria cases and deaths, amounting to 213 million and 94% respectively 2. Several species of mosquitoes belonging to the genus Anopheles are known to transmit malaria. In the African continent, Anopheles gambiae, An. coluzzii, An. arabiensis and the An. funestus were reported to be the major malaria vectors 3. Other members of the An. gambiae complex includes An. bwambae, An. melas, An. merus, An. quadriannulatus and An. amharicus. All were noted to exhibitgenetic differences, behavioural and ecological that can affect their vectorial capacity 4. Over the years, vector control has contributed immensely to the management of malaria and other vector-borne infectious diseases 5. Several control measures are employed against the mosquitoes. The control measures are either targeted at the adults or the larvae. The control measures against the adults are majorly chemical-based used in either Long Lasting Insecticide Nets (LLINs) or as Indoor Residual Spray (IRS) [2]. Whereas the larvae are controlled biologically employing larvivorous fishes such as the Gambusia spp or through the use of toxic chemical formulations or plant extracts 6. The major constraint with the chemical-based control measures is the resistance development by the vectors. Successive usage of chemical-based insecticides over time resulted to the development as well as the widespread resistance in vector populations 7,3. Another challenge with chemical control reported is the potentiality of the insecticides to cause environmental and human health toxicity 8. Consequent to the failure of the aforementioned control strategies, the need of a novel and safer eco-friendly strategy is required to have effective control of the vector with minimal toxicity effect on the environment and human health. The emergence of nanotechnology is seen as a prospect for alternative vector control by many vector biologists. Nanotechnology deals with the synthesis and application of nanosized particles with configurations ranging from 1 to 100 nm in dimension. These particles are referred to as nanoparticles (NP) 9,7. Generally, due to their small size, nanoparticles can pass through the larvae’s body wall into the cell and alter the physiological function of the larvae which causes death 10. Ingestion of nanoparticles by larvae and absorption of nanoparticles are two ways in which nanoparticles penetrate the cuticle cells 10. These are referred to as mechanisms of action of nanoparticles against mosquito larvae. Findings have shown that there is limited proof of toxicity towards non-target organisms of nanoparticles synthesized through the green route against mosquito larvae 11. For instance, 12 reported no toxicity effect of the silver nanoparticles synthesized using Vinca rosea– synthesized against P. reticulata, when exposed to the dosages toxic against Anopheles stephensi and the Cx. quinquefasciatus for 72 hours. In the same way, 13 reported that there was no detection of toxicity of the silver nanoparticles synthesized using the dried green fruit of Drypetes roxburghii against the insect P. reticulata, after exposure for 48 hours to LC50 of the 4th instars of Anopheles stephensi and Culex quinquefasciatus larvae. It was also reported that nanoparticles synthesized using Solanum nigrum berry extracts against the larvae of Anopheles stephensi and Culex quinquefasciatus were also toxic to some predators of mosquito (e.g. Toxorhynchites larvae and the Diplonychus annulatum) and larvae of Chironomus circumdatus 14. Nanoparticles and other inert dust were suggested to serve as alternative strategy to the current broad-spectrum insecticides used in the management of pest populations. This is recommended since most pests are developing resistant to the chemical-based conventional pesticides 15.
It was suggested that reliable data on the biological effects of nanoparticles and then the Physico-chemical behaviour of nanoparticles conditions provide a prospect for predicting the potential impacts of the nanoparticles in both environmental and biological systems 16. Nanoparticles are basically synthesized in three ways; chemical, physical and green synthesis. Among these, green synthesis accords more advantages of cost-effectiveness and Eco-friendliness 17. More so, the plant-mediated synthesis of nanoparticles has been proven to be a cheap, single step, requires less pressure, energy and temperature and uses less toxic chemicals in comparison to physical and chemical methods 18. Consequently, there has been a growing number of plant-based green syntheses of metal nanoparticles in recent times and their wide applications in different spheres in industry, agriculture and biology 19. Several reports indicated the toxicity of ZnO nanoparticles against microbes 20, fungi and mosquitoes 21. The toxic effect of SiO2 nanoparticles on insects, microbes and molluscs is also well documented 22,24. However, there are limited toxicity reports on composite containing ZnO NPs and SiO2 NPs against mosquito larvae. Gum Arabic is a hydrocolloid polysaccharide extracted from Acacia senegal var. the Senegal trees. Gum Arabic are non-toxic and are used as, stabilizing agents, film formers, flocculants, thickeners, suspending agents, emulsifiers and surfactants 24,25. Recently, the green sol-gel method with the aid of Gum Arabic has been reported for the synthesis of CuO@SiO2 26 and NiO 27. Here, we have reported the novel synthesis and the characterization of ZnO@SiO2 nanocomposite using gum Arabic. The toxicity of the nanoparticles on the larvae of the Anopheles gambiae complexwas also revealed.
Experimental
Materials and Method
Chemicals and apparatus/instrument
All the reagents used in this study are analytical grade and DBH products. All the glassware and apparatus used in this study are pyrex products. For this research the following apparatus and chemicals were used: Zn(NO3)2.6H2O, Silica gel, distilled water, Gum Arabic, mortar and the pestle, conical flasks, beakers, test tubes, Whatman filter paper, watch glass, measuring cylinder, oven, funnels, hot plate, petri dish, weighing balance, cotton wool, masking tape, spatula, glass rod stirrer, aluminium foil paper, syringes, Furnace, Ultraviolet-Visible spectrophotometer (model 6705), micropipette, FT-IR (PerkinElmer Spectrum Version 10.0309) and SEM/EDX(model PhenomWorld).
Collection of Gum Arabic Extrudes
A fresh Acacia senegalensis extrudewas obtained from Billiri, a Local Government Area in Gombe state. The extrude was ground using mortar and pestle until it becomes powder. It was kept under room temperature [as reported by 26] in the laboratory of the Department of Chemistry, Gombe State University.
Synthesis of ZnO@SiO2 nanocomposite
The synthesis of the nanocomposite followed the prescription of 28. A beaker containing 40ml of 1g of Arabic gum was stirred for 10 minutes at the temperature of 90°C on a hotplate for complete dissolution. Following this, 2g of Zinc nitrate and 2g of the Silica gel were then added and stirred for 120 minutes and the same temperature was maintained. By the addition of Copper nitrate and the Silica, the solution was changed colour to blue-green. Subsequently, the solution becomes viscous. Cloudy formation at the bottom of the beaker indicates that resin is formed. Resin obtained was then transferred into crucible and covered with paper foil and then placed in the laboratory furnace at 450oC for 2 hours to get ZnO@SiO2 Nanocomposite powder. The SiO2 was also calcined at 450oC for 2 hours.
Characterization of ZnO@SiO2 Nanocomposite, SiO2 and Gum Arabic
The following techniques were used for the characterization: Fourier-Transform Infrared Spectrophotometer (FTIR), The Scanning Electron Microscopy (SEM), UV-Visible spectrophotometer, The Dynamic Light Scattering (DLS), and X-Ray Fluorescence (XRF).
Collection of Anopheles gambiae complex
The laboratory-reared larvae of Anopheles gambiae complex were obtained from the Gombe State Roll Back Malaria (Malaria Control Booster Insectary, Gombe, Nigeria) identified using 29. The larvae were kept in and maintained at 25 ± 20C and were stored in a dechlorinated water. A mixture of low-fat biscuit and yeast powder in the ratio of 3:1 was used for feeding the larvae as per 30.
Toxicity test of ZnO@SiO2 against Anopheles gambiae complex
To prepare the stock solution, 0.1g of the ZnO@SiO2 nanocomposite was measured and then diluted in distilled water using a 1000 ml volumetric flask and was shaken to obtain a 100 mg/L concentration. Then the toxicity of ZnO@SiO2 againstthe larvae of Anopheles gambiae complex was assessed following [30] as per the method of 31, with minor modifications. Twenty-five larvae were then placed in 200 ml of the de-chlorinated water in a glass beaker (500 ml), and then 1 ml of the desired concentration of ZnO@SiO2nanocomposite was then added (10mg/L, 20mg/L, and 25mg/L). Each concentration was tested against each of the larval instar accordingly, with control in each case containing distilled water. The percentage mortality was computed as follows:

Statistical analysis
The average mortalities were calculated from the replicate and represented in percentages. The LC50 and LC90 were also computed. SPSS (Statistical software package) version 25.0 was used.
Result/Discussion
Toxicity test
The toxicity studies were carried out for 24 hours on the four larval instars of the Malaria vectors (1st, 2nd, 3rd, and 4th). The larval instars were tested at various concentrations of 10, 20 and 25 mg/L of the nanocomposite (Table I). The percentage toxicity of 1st instar larvae at concentrations of 10, 20 and 25mg/L were 50±11.31371, 65±7.071068 and 65±7.071068% respectively. The 2nd instar showed 40±2.828427, 55±7.071068 and 60±7.071068% mortality respectively when tested with concentrations of 10, 20, and 25mg/L of the nanocomposite. The percentage mortality for 10, 20 and 25mg/L concentrations were 40±2.828427, 55±7.071068 and 60±7.071068% respectively while the 4th instar indicated 35±4.242641, 50±7.071068, 60±2.828427% mortality for the respective concentrations. 32 reported variable toxicity between different kinds of nano-silica in larval toxicity test of An. stephensi, Ae. aegypti, and Cx. quinquefasciatus. The results were shown to be in the range of 93-100% mortality in 48 hours. The variation of this finding may be attributed to the fact that the nanoparticles in their study used one metal whereas in the present study, two different metals were used, Moreso, the method of the synthesis vary. Notably, the juvenile instars proved to be more susceptible to the nanocomposite just as reported by; [14, 32]. The LC50 for the 1st, 2nd, 3rd and 4th instars were found to be 9.11, 15.85, 15.85 and 18.288 mg/L respectively whilst the LC90 for the 1st, 2nd, 3rd and 4th instars were found to be 157.254, 157.254, 157.254 and 126.132mg/L respectively.
Table 1: Toxicity status of ZnO@SiO2nanocomposite against first to fourth instars larvae of Anopheles gambiae complex.
Life cycle |
Conc.(mg/L) |
% Mortality and SD |
% Control mortality |
LC50 |
LC90 |
χ2 |
r |
1st Instar |
10 20 25 |
50±11.31371 65±7.071068 65±7.071068 |
0.00 0.00 0.00 |
9.11 |
157.254 |
0.084 |
0.945 |
2nd Instar |
10 20 25 |
40±2.828427 55±7.071068 60±7.071068 |
0.00 0.00 0.00 |
15.85 |
157.254 |
0.00 |
0.999 |
3rd Instar |
10 20 25 |
40±2.828427 55±7.071068 60±7.071068 |
0.00 0.00 0.00 |
15.85 |
157.254 |
0.00 |
0.999 |
4th Instar |
10 20 25 |
35±4.242641 50±7.071068 60±2.828427 |
0.00 0.00 0.00 |
18.288 |
126.132 |
0.092 |
0.997 |
LC50= Lethal concentration that kills upto 50% of the larvae exposed,
LC90= Lethal concentration that kills upto 90% of the larvae exposed,
χ2=chi square value, r = correlation coefficient; SD= Standard deviation
Ultraviolet-visible analysis
The absorption spectrum of the synthesized ZnO@SiO2 nanocomposite at different wavelengths ranging between 260 and 380 nm disclose the maximum absorption wavelength at 280 nm, (Figure 1). The maximum absorption wavelength of 300 nm was reported for CaO@SiO2 nanocomposite by 33. The optical property reveals that they fall within the same range as the present study.
![]() |
Figure 1: The UV-Visible spectrum for ZnO@SiO2 nanocomposite. |
FT-IR Analysis
The FT-IR spectrum of Gum Arabic, SiO2 and ZnO@SiO2 nanocomposite is shown in Figures 2, 3 and 4 respectively. The prominent peaks for Gum Arabic are 3242.8, 2933.4, 1606.5, 1416.4 and 1200-900 cm−1 which represents; O-H stretching of the glucosidic ring, the C-H stretching, the COO-symmetric stretching, COO-asymmetric stretching, and fingerprint of carbohydrate respectively. For SiO2, the band at the 1067.44 cm-1 conform to the asymmetric stretching vibration of Si-O-Si bond. While peaks at 972.8 and 797.7 cm-1 correspond to Si-OH bond as reported by several findings 34-37. The peak at 3753.4-3403.1 cm-1 denotes H-O-H stretching mode (for the silanol group and the adsorbed water) [34-38]. For ZnO@SiO2 nanocomposite, almost peaks in the spectrum of SiO2 and Gum Arabic remains except the new peak at 670.9 cm−1 which correspond to Zn-O and Si-O bond 34-38. Thus, the formation of ZnO@SiO2 nanocomposite was confirmed.
![]() |
Figure 2: FT-IR spectrum of Gum Arabic. |
![]() |
Figure 3: The FT-IR spectrum of SiO2 |
![]() |
Figure 4: The FT-IR spectrum of ZnO@SiO2 Nanocomposite. |
Scanning Electron Microscopy (SEM) and Dynamic Light Scattering (DLS) Analysis
Figure 5A and Figure 5B shows the SEM image of ZnO@SiO2 nanocomposite and SiO2 respectively. The image from SEM showed large irregular particles and the coating of the silica on the ZnO is seen when compared to the SEM image of silica. Also, the SEM image of SiO2 showed irregular large particles, (Figure 5B).
The measurement of the particle sizes were done using Zetasizer (Malvern Instrument Ltd, Worcestershire, UK) at 25o C based on the laser Doppler velocimetry and the DLS techniques. The DLS gives the polydispersity index (PDI), which suggests the width of the particle size distribution, is calculated thus: the square root of peak (standard deviation/average size). PDI < 0.1 suggests the sample is monodisperse while PDI > 0.1 indicate the sample is polydispersed. If When PDI is lies between 0.1-0.4, the sample would then have a moderate polydispersed distribution of particle size. The PDI >0.4 shows that the sample would then have a wide particle size distribution. In this research, size distribution by intensity was found to be 88.1 nm (76.3%), 410.8 nm (20.9%) and 5387 nm (2.7%) for peak 1, peak 2 and peak 3 respectively, (Figure 6). The average size distribution was found to be 147.6 nm. The polydispersity index (PDI) for peak 1, peak 2 and peak 3 were found to be 0.5, 0.96 and 1.5 respectively. The polydispersity index (PDI) shows that the sample is polydispersed with a wide distribution of particle size. These findings correlate well with the SEM result.
![]() |
Figure 5: A; SEM image of ZnO@SiO2 nanocomposite, B; SEM image of SiO2 |
![]() |
Figure 6: DLS result for ZnO@SiO2 nanocomposite |
X-Ray Fluorescence (XRF) Analysis
The elemental composition of Gum Arabic, SiO2, and ZnO/SiO2 nanocomposite are presented in Table 2. Various elements with a different percentage such as Si, Zn, Al, O2, S, Cl, Ca, Ti, K, V, Cr, Fe, Co, Mn, Ni, Cu, Nb, Zr, Mo, W and Ag were found in both Gum Arabic and SiO2 sample. Gum Arabic has been earlier reported to contain Al, Ca, Ba, Fe, Mg, K, Mn, P, Sr and S 39. The elemental composition of the nanocomposite almost followed the same trend with the precursors (Gum Arabic and SiO2). This indicates that other impurities in the nanocomposite are from the precursors (Gum Arabic and SiO2). The components composition of SiO2 and ZnO/SiO2 nanocomposite are presented in Table 3. For SiO2 components, SiO2 contains 97.361 % whereas other components made up 2.639 %. For the ZnO/SiO2 nanocomposite, ZnO is 42.068 % and SiO2 is 44.794 %. Overall, the nanocomposite is made up of 86.862 % while other components are 13.138 %.
Table 2: Elemental composition of Gum Arabic, SiO2, and ZnO/SiO2
S/N |
Elements |
Gum Arabic (wt. %) |
SiO2 (wt. %) |
ZnO/SiO2 (wt. %) |
1 |
O |
29.508 |
52.641 |
35.661 |
2 |
Si |
3.090 |
45.511 |
20.939 |
3 |
Zn |
0.174 |
0.002 |
33.796 |
4 |
Mg |
6.831 |
– |
– |
5 |
Al |
5.236 |
0.766 |
1.678 |
6 |
S |
0.219 |
– |
0.062 |
7 |
Cl |
4.288 |
0.783 |
0.906 |
8 |
K |
16.450 |
– |
1.188 |
9 |
Ca |
27.077 |
0.142 |
1.297 |
10 |
Ti |
0.284 |
0.013 |
0.017 |
11 |
V |
0.060 |
0.004 |
0.010 |
12 |
Cr |
0.068 |
0.012 |
0.015 |
13 |
Mn |
4.172 |
0.016 |
0.106 |
14 |
Fe |
1.034 |
0.032 |
0.050 |
15 |
Co |
0.113 |
– |
0.001 |
16 |
Ni |
0.013 |
0.003 |
– |
17 |
Cu |
0.828 |
0.028 |
0.019 |
18 |
Zr |
0.011 |
0.004 |
0.250 |
19 |
Nb |
0.172 |
0.003 |
2.955 |
20 |
Mo |
0.056 |
0.001 |
– |
21 |
Ag |
0.226 |
0.005 |
– |
22 |
Ba |
– |
0.021 |
0.052 |
23 |
Ta |
– |
0.007 |
0.324 |
24 |
W |
0.049 |
0.004 |
0.674 |
Table 3: Compounds composition of SiO2, and ZnO/SiO2
S/N |
Components |
SiO2 (wt. %) |
ZnO/SiO2 (wt. %) |
1 |
SiO2 |
97.361 |
44.794 |
2 |
ZnO |
0.002 |
42.068 |
3 |
Al2O3 |
1.448 |
3.171 |
4 |
K2O |
– |
1.431 |
5 |
CaO |
0.198 |
1.815 |
6 |
Nb2O3 |
0.004 |
3.719 |
7 |
Cr2O3 |
0.018 |
0.022 |
8 |
V2O5 |
0.007 |
0.019 |
9 |
MnO |
0.021 |
0.136 |
10 |
Fe2O3 |
0.046 |
0.072 |
12 |
CuO |
0.035 |
0.023 |
13 |
MoO3 |
0.002 |
– |
14 |
WO3 |
0.005 |
0.850 |
15 |
SO3 |
– |
0.154 |
16 |
BaO |
0.024 |
0.058 |
17 |
Ta2O5 |
0.008 |
0.395 |
18 |
TiO2 |
0.022 |
0.029 |
19 |
Cl |
0.783 |
0.906 |
20 |
ZrO2 |
0.005 |
0.337 |
21 |
NiO |
0.004 |
– |
22 |
Ag2O |
0.005 |
– |
Mechanism of formation of ZnO/SiO2 NPs using green sol-gel method
The chains of Arabic gum which are larger are polysaccharides that are natural, forming complexes with Zn2+ ions that interact with functional groups like –COOH, –OH and –NH2 during gel formation 40. The gel complexes formed lead to nucleation and growth of ZnO NPs. The simple explanation for the mechanism of the formation and the growth of ZnO in the presence of the Arabic gum and Silica is illustrated thus:
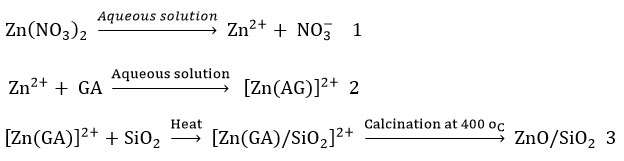
Conclusion
This work reported the novel synthesis of ZnO@SiO2 nanocomposites using gum Arabic. SEM, XRF, UV-spectrophotometric analysis, DLS and FT-IR were employed for characterization of the nanocomposite. The toxicity of the nanocomposite against the larval instars of malaria vectors was tested and the LC50 and LC90 were computed. The ZnO@SiO2 nanocomposites could be a potential larvicide for the control of malaria vectors.
Acknowledgement
We appreciate the Roll Back Malaria, Gombe State Project and the Technologist at the Chemistry and Zoology Laboratories, Gombe State University for their support. We also acknowledge the staff of the Department of Chemical Engineering, Ahmadu Bello University (ABU) Zaria, Nigeria for the characterization of the nanoparticles.
Conflict of Interest
There was no conflict of interest between the authors.
Funding Sources
There is no funding source.
References
- W.H.O. 2018, World Malaria Report. World Health Organization, Geneva, Switzerland. http://apps.who.int/iris/bitstream/handle/ 10665/275867/9789241565653-eng.pdf
- W.H.O. 2019, World Malaria Report. World Health Organization, Geneva, Switzerland. 1-232. https://www.who.int/publications/ i/item/9789241565721
- Oduola, A. O.; Abba, E.; O. Adelaja, O.; Ande A. T.; Yoriyo, K. P.; Awolola T. S. J of Arthropod-Borne Dis., 2019, 13, 50-61. Doi: 10.18502/jad.v13i1.932
CrossRef - Fontenille, D.;Cohuet, A.; Awono-Ambene, P. H.; Antonio-Nkondjio, C.;Wondji, C.; Kengne, P.;Dia, I.;Boccolini, D.,Duchemin, J. B.;Rajaonarivelo, V.;Dabire, R.; Adja-Akre, M.;Ceainu, C.; Le Goff, G.; Simard, F.Medicine Tropicale (Mars)., 2003,63, 247-253. https://pubmed.ncbi.nlm.nih.gov/14579461/
- Neafsey, D.E.; Waterhouse,R.M. Abai,M.R.;Aganezov,S.S.; Alekseyev,M.A.; Allen, J. E. et al.Sci.,2015,347, 1258522-1258522. Doi: 10.1126/science.1258522
CrossRef - Mehlhorn, H.; Encyclopedia of Parasitology, fourth ed. Springer, New York., 2008, p. 893. https://www.springer.com/gp/book/9783662439777
- Hamed, B.; Zahra, A.; Mohammad, T. R.; Aleksandra, B.; Maraolo, A. E.; Robertson, L. J.; Masjedi, A.; Shahrivar, F.; Ahmadpour, E. Nanomed., 2019, 18, 221-233. Doi:10.1016/j.nano.2019.02.017
CrossRef - Tennyson, S., Ravindran, K. J.; Arivoli, S. Asian Pac. J. Trop. Biomed., 2012, S1130- S1134. Doi:10.1016/S2221-1691(12)60372-4
CrossRef - Honary, S.; Barabadi, H.; Ebrahimi, P.; Naghibi, F.; Alizadeh, A. J Nano Res., 2015, 30, 106-115. Doi:10.4028/www.scientific.net/JNanoR.30.106
CrossRef - Hatem, F.; Li, H.; Dawood, H.; Jiqian, W.; Ghulam, A.; Hassan, G.; Mo, J. Nanomed. Biotech., 2018, 46, 558-567. Doi:10.1080/21691401. 2017.1329739
CrossRef - Benelli, G. Parasitol Res., 2016, 115, 23-34. Doi: 10.1007/s00436-015-4800-9
CrossRef - Subarani, S.; Sabhanayakam S.; Kamaraj, C. Parasitol. Res., 2013,112, 487-499. Doi: 10.1007/s00436-012-3158-5
CrossRef - Haldar, K. M.; Haldar, B.; Chandra, G. Parasitol Res., 2013, 112, 1451-1459. Doi: 10.1007/s00436-013-3288-4
CrossRef - Rawani, A; Ghosh, A.; Chandra, G. Acta Tropica., 2013, 128, 613-622. Doi: 10.1016/j.actatropica.2013.09.007
CrossRef - Jin, Z.; Liang, Q.; Liang, Y.; Tan, X.; Guan, Ormesher L. Proceedings of the 7th International Working Conference on Stored-product Protection; 1999 Oct. 14–19, Beijing. Chengdu (China): Sichuan Publishing House of Science and Technology, 738. http://spiru.cgahr.ksu.edu/ proj/iwcspp/iwcspp7.html
- Angela, I.; Katre, J.; Olesja, B.; Monika, M.; Villem, A.; Kasemets, K.; Blinova, I.; Heinlaan, M.; Slaveykova, V.; Kahru, A. Nanotoxicol., 2014, 8,57-71. Doi: 10.3109/17435390.2013.855831
CrossRef - Ravindra, B. M.; Seema, L. N.; Neelambika, T. M.; Gangadhar, S. M.; Nataraja. K.; Kumar, S. V.; Res Biotechnol., 2012, 3,26-38.
https://updatepublishing.com/journal/index.php/rib/article/download/2420/2398 - Kumar, S.; Viney, L.; Deepti, P.; Nanomed., 2015, 1-21. Doi:10.2217/nnm.15.112
CrossRef - Rajan, R.; Chandran, K.; Harper, S. L.; Yun, S. I.; Kalaichelvan, P. T. Ind Crops Prod., 2015, 70, 356-373. Doi:10.1016/j.indcrop.2015.03.015
CrossRef - Siddiqi, K. S.; Ur Rahman, A.; Tajuddin, A.; Husen, A. Nanoscale Res. Lett., 2018, 13, 1-13. Doi: 10.1186/s11671-018-2532-3
CrossRef - Naif, A. A.; Mariadhas, V. A. Nanomater, 2018, 8, 500-512. Doi:10.3390/nano8070500
CrossRef - Ziaee, M.; Ganji, Z. J. Plant Prot. Res., 2016, 56, 250-256. Doi: 10.1515/jppr-2016-0037
CrossRef - Reddla, H .B.; Pulicherla, Y.; Nataru, S. Bull. Mater. Sci., 2018, 41, 1-8. Doi:10.1007/s12034-018-1584-4
CrossRef - Venkatesham, M.; Ayodhya, D.; Madhusudhan, A.; Veerabhadram, G. Int. J. Green Nanotech., 2012, 4, 199-206. Doi:10.1080/19430892. 2012.705999
CrossRef - Daoub, R. M. A.; Elmubarak, A. H.; Misran, M.; Hassan, E. A.; Osman, M. E. J Saudi Soc. Agric. Sci., 2018, 17,1-24. Doi:10.1016/j.jssas.2016.05.002
CrossRef - Abba, E.; Shehu, Z.; Yoriyo, K. P.; Lamayi, D. W.; Ayuk, C. N.; Abubakar, Z. A.; Kambel, R. D. Plant Cell Biotechnol. Mol. Bio., 2021, 22, 99-108. https://www.ikprress.org/index.php/PCBMB/article/view/6684
- Sabouri, Z.; Akbari, A.; Hosseini, H. A.; Khatami M.; Darroudi, M. Green Chem. Let. Rev., 2021, 14, 404-414. Doi: 10.1080/17518253.2021.1923824
CrossRef - Fardood, S. T.; Ramazani, A.; Moradi, S. Chem. J. of Moldova., 2017, 12,115-118. Doi:10.19261/cjm.2017.383
CrossRef - Gillies, M. T.; Coetzee, M. A Publication of the South African Institute for Medical Research, 1987, 55, 1-282. http://mosquito-taxonomic-inventory.info/supplement-anophelinae-africa-south-sahara-afrotropical-region
- W.H.O. 2005, Guidelines for Laboratory and Field Testing of Mosquito Larvicides; WHO/CDS/WHOPES/ GCDPP/2005.13; World Health Organization: Geneva, Switzerland. 1-41. https://apps.who.int/ iris/handle/10665/69101
- Rajakumar, G.; Abdul Rahuman, A. ActaTropica., 2011, 118, 196-203. Doi:10.1016/j.actatropica.2011.03.003
CrossRef - Tapan, K. B.; Raghavendra, K.; Arunava, G. Parasitol. Res., 2012, 111, 1075-1083. Doi: 10.1007/s00436-012-2934-6
CrossRef - Wilson, L. D.; Mela Y.; Zaccheus, S.; Yakong D.M. and Sani I.A. J. Mater Sci. Res. and Rev., 2019, 4, 1-7. https://journaljmsrr.com/ index.php/JMSRR/article/view/30116/56510
- Aymen, H.; Abdelali, M.; Ouanassa, G.; Mohamed, G. Int. J. Eng Appl. Sci., 2018, 5, 23-28. https://www.ijeas.org/download_data/ IJEAS0502013.pdf
- Subramaniyan, A.; Visweswaran, V.; Saravana, K. C.; Sornakumar, T. Nanochem. Res., 2018, 3,79-84. Doi: 10.22036/ncr.2018.01.008
- Yoon, C.; Jeon, B.; Yoon, G. Appl. Sci., 2018, 8,1-11. Doi:10.3390/app8071127
CrossRef - Mohamed, R.M.; Aazam, E. S. Desalination and Water Treatment, 2012, 50, 140-146. Doi:10.1080/19443994.2012.708559
CrossRef - Samaneh, L. Mohammad, G. Surf. Coatings Tech., 2017, 310, 129-133. Doi:10.1016/j.surfcoat.2016.12.032
CrossRef - Mahendran, T. P. A.; Williams, G. O.; Phillips, S.; Al-Assaf, S.; Baldwin, T. C. J. Agric Food Chem., 2008, 8: 56, 9269-9276. Doi: 10.1021/jf800849a
CrossRef - Ba-Abbad, M. M.; Mohammada, A. W.; Takriffa, M. S.; Rohania, R.; Mahmoudi E.; Faneera, K. A.; Benamo, A. Chem. Eng. Transactions, 2017, 56,1699-1704. Doi: 10.3303/CET1756284
This work is licensed under a Creative Commons Attribution 4.0 International License.