Reactions of MoCl5 with 4-Methylpyridine, 2-Methylpyridine and 1-Methylimidazole in Tetrahydrofuran
1Punjab Technical University, Kapurthala, India.
2Department of Applied Chemistry, Giani Zail Singh Campus College of Engineering and Technology, Dabwali Road, MRSPTU Bathinda-151001-India.
Corresponding Author E-mail: gursharans82@gmail.com
DOI : http://dx.doi.org/10.13005/ojc/370523
Article Received on : 06-Aug-2021
Article Accepted on :
Article Published : 20 Oct 2021
Reviewed by: Dr. Femina Felix

Second Review by: Dr.Kamatchi Selvaraj

Final Approval by: Dr. Ioana stanciu
MoCl5 reactions with 4-methylpyridine/2-methylpyridine/1-methylimidazole in THF in 1:1/1:2 stoichiometric ratios, at room temperature were carried out. The following products were synthesized: MoO2Cl(C6H7N), 1;
Mo2O2Cl5(C6H7N)2(C4H8O)2,2; Mo4O4Cl4(C6H7N)3(C4H8O)2, 3 and Mo2O4Cl4(C4H6N)2(C4H8O), 4. These compounds have been investigated by FT-IR (transmission mode), FT-1H NMR, FT -13C NMR, microbiological, LC-MS and elemental (C, H, N, Mo, Cl) studies. In view of the sensitivity of all the reactants and products towards oxidation/hydrolysis by air/moisture, all the reactions and products were handled using dry nitrogen atmosphere in vacuum line. LC-MS and elemental studies agree with the formulae of compounds.
4-Methylpyridine; 2-Methylpyridine; 1-Methylimidazole; MoCl5; Microbiological
Download this article as:
Copy the following to cite this article: Kumar R, Singh G. Reactions of MoCl5 with 4-Methylpyridine, 2-Methylpyridine and 1-Methylimidazole in Tetrahydrofuran. Orient J Chem 2021;37(5). |
Copy the following to cite this URL: Kumar R, Singh G. Reactions of MoCl5 with 4-Methylpyridine, 2-Methylpyridine and 1-Methylimidazole in Tetrahydrofuran. Orient J Chem 2021;37(5).Available from: https://bit.ly/3pnvFPd |
Introduction
Pyridine derivatives have a number of biological activities, like antimycobacterial1, antimicrobial2, 3, analgesic4, anticonvulsant5, antitumoral6, antimalarial7, antiparkinsonian8, cytotoxic9, pesticidal10, antidiabetic11, antibacterial12, inhibitory13 and receptor14 antagonists. Electron donating groups15 in pyridine derivatives increase the biological activity of transition metal-pyridine derivative compounds.
4-Methylpyridine
4-Methylpyridine16 is used as a precursor for the preparation of a number of heterocyclic compounds. It is used for the preparation of many compounds of medicinal interest, like ‘isoniazid’ used as antituberculosis drug17.
2-Methylpyridine
2-Methylpyridine18 acts as an intermediate for the manufacture of drugs, like picoplatin, amprolium, encainide and dimethindene. It is used as a precursor for the preparation of 2-vinylpyridine. It is used to manufacture nitrapyrin19 which controls loss of NH3 from fertilizers. It is also used to prepare herbicide piclorand.
1-Methylimidazole
Azoles20 are widely used in antibiotics and antifungal agents, because cytochrome P450 sterol 14α-demethylase of the pathogens are inhibited by them. Azole action mechanism involves,
Coordination of imidazole non-protonated nitrogen and enzyme heme-iron
π-cation interaction
Hydrogen binding
Only a few antifungal agents are available: azoles, allylamines, polyenes, fluoropyrimidines and thiocarbamates21. Among these agents, azoles are the most important because of their high therapeutic index. Effectiveness of the azole drug depends on the strength of the coordinate bond22.
Imidazole drugs have many applications23-35 as antifungal, 20-carboxypeptidase inhibitors, hemeoxygenase inhibitors, anticancer, anti-inflammatory, antibacterial, antimalarial, antitubercular, antiviral agents. They are also anticoagulants, antidiabetic HETE (20-Hydroxy-5, 8, 11, 14-eicosatetraenoic acid) synthase inhibitors, β-lactamase inhibitors and antiaging agents.
Metal chelates drugs are more effective than ligands themselves36-41.
Aim of Investigation
MoCl5 reactions with imides, diaminoalkanes, aromatic azoles, thiols like 4-phenylimidazole-2-thiol, 2-thiazoline-2-thiol, alkylpyridines and mercaptopyridine-N-oxide have been reported42-51 by the author.
Since applications of transition metal compounds with pyridine and imidazole derivatives are widening very fast, so MoCl5 compounds of 4-methylpyridine, 2-methylpyridine and 1-methylimidazole have been prepared and investigated. These complexes were studied with elemental analysis, FT-IR, LC-MS, FT-1H NMR, FT-13C NMR and microbiological studies.
Materials and Methods
4-Methylpyridine, 2-methylpyridine, 1-methylimidazole and MoCl5 used were of Sigma-Aldrich
Reactants and products being prone to oxidation by air/moisture, so all reactions were carried out and products handled in vacuum line in dry nitrogen atmosphere.
4-Methylpyridine/2-methylpyridine/1-methylimidazole was added to the dropping funnel and dissolved in 10 ml dry THF. It was added dropwise to MoCl5 solution in THF with continuous agitation in 100 ml B-14 flask. Contents were stirred for 7-8 h. Filtration was carried out. Products from both the residue and filtrate were isolated and studied.
Molybdenum was estimated using 8-hydroxyquinoline gravimetrically52. Chlorine was estimated using silver nitrate gravimetrically52. Other elements were determined quantitatively by Thermo Finnigan Elemental Analyser. Proton/13C nuclear magnetic resonance spectra were recorded in DMSO-d6 with Multinuclear Brucker Avance-II 400 NMR spectrometer. Vibrational peaks have been studied using Perkin-Elmer 400 FTIR Spectrometer.LC-MS studies have been carried out in the span 0 – 1100 m/z. P. U. Chandigarh, India provided the instrumental support.
Molybdenum compounds activity against bacteria and fungi was investigated by ‘Agar Well Diffusion Assay’ method. Various strains used are: fungi Candida albicans and Aspergillus niger, Gram positive bacteria Staphylococcus aureus and Gram negative bacteria E. coli. The Microbial Type Culture Collection and Gene Bank, Chandigarh, India cultures were used. Amoxicillin and ketoconazole for bacteria and virus, respectively were used as standard drugs for comparison. Studies were performed at Indo Soviet Friendship College of Pharmacy, Moga, Punjab, India.
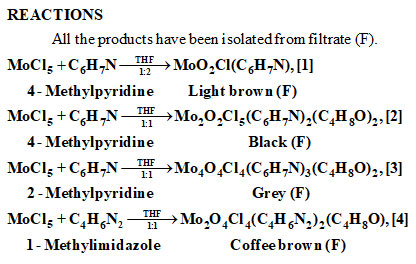
Results and Discussions
Elemental Studies
Table 1: (observed (theoretical) percentage)
Compounds |
Chlorine |
Molybdenum |
Hydrogen |
Carbon |
Nitrogen |
MoO2Cl(C6H7N), [1] (Light brown/256.5) |
13.74 (13.84) |
36.96 (37.42) |
03.13 (02.72) |
27.65 (28.07) |
04.85 (05.45) |
Mo2O2Cl5(C6H7N)2(C4H8O)2, [2] (Black/731.5) |
23.57 (24.26) |
25.53 (26.24) |
04.00 (04.10) |
31.93 (32.80) |
03.16 (03.82) |
Mo4O4Cl4(C6H7N)3(C4H8O)2, [3] (Grey/1013.0) |
13.23 (14.01) |
37.11 (37.90) |
03.82 (03.65) |
30.09 (30.79) |
03.27 (04.14) |
Mo2O4Cl4(C4H6N2)2(C4H8O), [4] (Coffee brown/634.0) |
21.78 (22.39) |
29.67 (30.28) |
03.09 (03.15) |
21.92 (22.71) |
07.13 (08.83) |
FT-IR Spectra
Ring υ(C-H) of 4-methylpyridine50, 53-60 occurs at 3072 cm-1 and the corresponding str. in 1 has been noted at 3090 cm-1. Ring υ(C=N) and torsion show upward shift, but ring δ(C-H) shows downward shift, showing Mo(dπ)→N(pπ). 976 cm-1 peak suggests the presence of terminal Mo=O45, 61, 62 in [1]. υ(Mo=O) decreases on N→Mo coordination63. Coordinate bond and Mo=O are trans to each other. Molybdenum abstracts64, 65 oxygen from THF to form Mo=O (Table-2).
Ring υ(C-H) has not been noticed in 2. It has been observed that ring υ(C=N) and torsion show upward shift, but ring δ(C-H) has decreased, as a result of Mo(dπ)→N(pπ). 975 cm-1 str. of Mo=O45, 61, 62 group at terminal position is observed. υ(Mo=O) decreases on N→Mo coordinate bond63 formation. Coordinate bond is trans to Mo=O. Molybdenum abstracts64, 65 oxygen from THF forming Mo=O (Table-2).
Table 2: (FT-IR absorptions in cm-1 )
Assignments |
4-Methylpyridine50, 53-60 |
[1] |
[2] |
Ring υ(C-H) |
3072, 3034 |
3403 b, 3090 sh |
3399 s |
Methyl υ(C-H) |
2990, 2927 |
2951 sh |
|
Ring υ(C=N) |
1611, 1564 |
1640 s |
1640 s |
Methyl δasym(C-H) |
1499 |
1507 m |
1508 w |
Ring δ(C-H) |
1458, 1445, 1418, 1365, 1194 |
1445 sh, 1316 w |
|
Methyl δsym(C-H) |
1383 |
1380 w |
1381 w |
Ring υ(C-N) |
1227 |
1256 w |
|
υ(C-CH3) |
1210 |
1203 w |
1204 sh |
Methyl ρ(C-H) |
1114 |
1099 w |
1111 sh |
Ring breathing |
1072 |
1039 w |
|
Ring out of plane bending |
994, 877 |
870 sh |
|
Ring ꞷ(C-H), ꞷ(C-C), ꞷ(C-N) |
730 |
794 m, 734 m |
791 w, 735 w |
Ring torsion |
524 |
619 sh , 566 w, 521 w |
570 w |
δ(C-CH3) |
486 |
476 w |
476 w |
Terminal Mo=O45, 61, 62 str. |
976 s |
975 m |
Ring υ(C-H) in 2-methylpyridine58, 59, 66, 67 is noted at 3065 cm-1, but [3] does not show this vibration. υ(C=N) in [3] has been noted at 1632 cm-1 showing an upward shift47 by 36 cm-1 and torsion was observed at 571 cm-1 showing an upward shift47 by 24 cm-1. This upshift is because of Mo(dπ)→N(pπ). υ(Mo=O) at 979 cm-1 in [3] has been recorded because of Mo=O45,61, 62 group at terminal position. υ(Mo=O) drops N→Mo coordination63. Coordinate bond and Mo=O are trans to each other. Molybdenum abstracts64, 65 oxygen from THF to form Mo=O (Table-3).
Table 3: (FT-IR absorptions in cm−1)
Assignments |
2-Methylpyridine58, 59, 66, 67 |
[3] |
Ring υ(C-H) |
3135 m, 3087 m, 3065 m |
3379 s |
Methyl υ(C-H) |
2960 m |
|
Ring υ(C=N) |
1596 vs |
1632 s |
Methyl δasym(C-H) |
1463 s |
|
Ring δ(C-H) |
1475 s, 1148 m, |
1477 sh |
Methyl δsym(C-H) |
1375 w |
1404 |
Ring υ(C-N) |
1297 s, |
1297 w |
υ(C-CH3) |
1235 m |
|
Methyl ρ(C-H) |
1075 |
|
Ring breathing |
1059 s |
1050 w |
Ring ꞷ(C-H), ꞷ(C-C), ꞷ(C-N) |
750 vs, 730 m |
762 s |
Ring ꞷ(C-C) |
630 m |
|
Ring torsion |
547 w |
571 sh |
δ(C-CH3) |
471 |
|
Terminal Mo=O45, 61, 62 str. |
|
979 s |
Unprotonated nitrogen of 1-methylimidazole68 coordinates with molybdenum. Ring υ(C=C) has been noted at 1553 cm-1 showing an increase of 36 cm-1. Ring υ(N-C) is located at 1446 cm-1 showing an increase of 39 cm-1. This increase in wave numbers on N→Mo coordination is caused by,
Inductive effect on ligand-molybdenum cation coordination,
dπ-pπ back bonding which changes the electron density on the ligand.
It causes electron density to increase in the ring which increases N→Mo coordinate bond strength. 978 cm-1 str. of Mo=O45, 61, 62 group at terminal position is observed. υ(Mo=O)
It causes electron density to increase in the ring which increases N→Mo coordinate bond strength. 978 cm-1 str. of Mo=O45, 61, 62 group at terminal position is observed. υ(Mo=O) decreases on N→Mo coordinate bond63 formation. Coordinate bond is trans to Mo=O. Molybdenum abstracts64, 65 oxygen from THF forming Mo=O (Table-4).
Table 4: (FT-IR absorptions in cm−1)
Assignments |
1-Methylimidazole68 |
[4] |
Ring υ(C-H) |
3017 m, 2955 w |
3398 s |
Ring υ(C=N) |
|
1630 s |
Ring υ(C=C) |
1519 vs |
1553 sh |
Ring υ(C-N) |
1409 m |
1446 w |
Ring δ(C-H) |
1107 m, 1087 m, 1035 vw |
1086 w |
Ring ꞷ(C-H), Ring τ(C-H) |
814 s, 774 s |
740 s |
Ring τ(C-H) |
640 s |
624 m |
ꞷ(N-H), Ring twisting |
525 |
569 m |
Terminal Mo=O45, 61, 62str. |
|
978 s |
FT-1H NMR Spectra
Compounds were dissolved in DMSO-d6 to take spectrum. DMSO-d669residual peak appears at 2.5 ppm.THF69 solution in DMSO-d6 shows CH2 peak at 1.7 ppm and O-CH2 peak at 3.6 ppm. ↑ and ↓ signify upfield/downfield shift.
When spectra of 4-methylpyridine50, 58, 59, 70 and 1 are compared, it is observed that absorptions show deshielding due to flow of π-electron density towards molybdenum cation on N→Mo coordinate bond formation (Fig. 1, Table-5).
![]() |
Figure 1: FT-1H NMR of MoO2Cl(C6H7N), [1] |
4-Methylpyridine50, 58, 59, 70 and 2 spectra on comparison show that absorptions go downfield in 2. This results from flow of π-electrons towards molybdenum on coordination (Fig. 2, Table-5).
![]() |
Figure 2: FT-1H NMR of Mo2O2Cl5(C6H7N)2(C4H8O)2, [2] |
Table 5: (1H-NMR absorptions in ppm)
Assignments |
4-Methylpyridine50, 58, 59, 70 in CDCl3 |
[1] |
[2] |
H-C2 & H-C6 (Ortho), |
8.4 2H d |
8.8 ↓ |
8.7 ↓ |
H-C3 & H-C5 (Meta), |
7.1 2H d |
7.8 ↓ |
7.9 ↓ |
CH3 attached to C4. |
2.3 3H s |
2.5 ↓ |
2.5 ↓ |
Residual69 DMSO-d6 |
2.5 |
2.5 |
|
THF69 O-CH2 |
3.5 |
||
THF69 CH2 |
1.7 |
2-Methylpyridine58, 59, 70 and [3] spectra reveal that all of the protons resonances occur at lower field due to delocalisation of ring π-electron density when ligand coordinates with molybdenum through nitrogen (Fig. 3, Table-6).
![]() |
Figure 3: FT-1H NMR of Mo4O4Cl4(C6H7N)3(C4H8O)2, [3] |
Table 6: (1H-NMR absorptions in ppm)
Assignments |
2-Methylpyridine58, 59, 70 in CDCl3 |
[3] |
CH3 attached to C2, |
2.5 3H s |
2.8 ↓ |
H-C5 (Meta), |
7.0 1H t |
7.9 ↓ |
H-C3 (Meta), |
7.1 1H d |
7.9 ↓ |
H-C6 (Ortho), |
8.4 1H d |
8.8 ↓ |
H-C4 (Para). |
7.5 1H t |
8.5 ↓ |
Residual69 DMSO-d6 |
2.5 |
|
THF69 O-CH2 |
3.6 |
|
THF69 CH2 |
1.8 |
[4] spectrum shows that all the protons of the 1-methylimidazole67, 68, 71 have deshielded on account of flow of π-electrons towards molybdenum on coordination (Fig. 4, Table-7).
![]() |
Figure 4: FT-1H NMR of Mo2O4Cl4(C4H6N2)2(C4H8O), [4] |
Table 7: (1H-NMR absorptions in ppm)
Assignments |
1-Methylimidazole67, 68, 71 in D2O |
[4] |
H-C4 |
7.0 1H |
7.6 ↓ |
H-C5 |
7.0 1H |
7.6 ↓ |
H-C2 (between two N) |
7.5 1H |
9.1 ↓ |
CH3 attached to N |
3.6 3H |
3.8 ↓ |
Residual69 DMSO-d6 |
|
2.5 |
THF69 O-CH2 |
|
3.6 |
THF69 CH2 |
|
|
FT-13C NMR Spectra
Compounds were dissolved in DMSO-d6 to take spectrum. DMSO-d669residual peak occurs at 39.52±0.06 ppm.↑ and ↓ represent upfield/downfield shift.
Spectrum of [2] reveals that all carbons of 4-methylpyridine72 show resonances at lower field except meta carbons on ligand-metal coordination (Fig. 5, Table-8).
![]() |
Figure 5: 13C-NMR of Mo2O2Cl5(C6H7N)2(C4H8O)2, [5] |
Table 8: (13C NMR absorptions in ppm)
Assignments |
4-Methylpyridine72 in CDCl3 |
[2] |
C-2 C-6 (ortho) |
149.6 |
157.6 |
C-3 C-5 (meta) |
146.9 |
139.8 |
C-4 (para) |
124.6 |
127.5 |
CH3 attached to C-4 |
20.8 |
22.1 |
Residual69 DMSO-d6 |
|
39.4 |
Microbiological Activity
Potency of molybdenum compounds synthesized against Gram-positive bacteria Staphylococcus aureus, Gram-negative bacteria E. coli, fungi Candida albicans and Aspergillus niger has been investigated. Amoxicillin and ketoconazole have been used as reference drugs against bacteria and fungi, respectively. Efficiency of molybdenum compounds have been tested by ‘Zone of inhibition’ method73. Potency of molybdenum compounds has been found to be good to control bacteria and fungi (Table-9). It is to be noted that,
Compounds 1, 2, 3 and 4 are more potent against E. coli than amoxicillin.
Compound 4 is more potent against C. albicans than ketoconazole.
Table 9: (MicrobiologicalStudy)
Compound (100 µg/ml) |
Zone of inhibition73 (mm) |
|||
S. aureus |
E. coli |
C. albicans |
A. niger |
|
Reference Drug |
24.99 |
17.85 |
21.37 |
27.88 |
[1] |
21.36 |
21.58 |
19.57 |
19.63 |
[2] |
21.28 |
23.14 |
19.87 |
18.54 |
[3] |
22.31 |
21.56 |
18.38 |
19.88 |
[4] |
19.87 |
21.32 |
22.17 |
21.38 |
Mass (LC-MS) Spectra
Masses of the most abundant isotopes have been used to calculate theoretical m/z values74 of the ions (Tables-10, 11),
![]() |
Table 10: LC-MS Ionization |
Table 11: (m/z values of ions)
Comp. |
Ion |
Calculated74 |
Observed |
Relative abundance |
[1] |
[C6H7N]+ |
93.05 |
94.06 |
100% |
[2] |
[MoO2Cl(C6H7N)]+ |
257.92 |
256.18 |
58% |
[MoO2Cl3(C6H7N)]+ |
327.85 |
328.25 |
3% |
|
[MoO2Cl3(C6H7N)]2+ |
163.92 |
166.14 |
2% |
|
[MoO2Cl3]+ |
234.80 |
238.21 |
4% |
|
[C6H7N]+ |
93.05 |
94.06 |
100% |
|
[3] |
[MoO2Cl(C6H7N)]+ |
257.92 |
256.15 |
92% |
[MoO2Cl3(C6H7N)]+ |
327.85 |
328.25 |
34% |
|
[MoO2Cl3(C6H7N)]2+ |
163.92 |
166.14 |
25% |
|
[C6H7N]+ |
93.05 |
94.06 |
100% |
|
[4] |
[MoOCl]+ |
148.86 |
149.05 |
1% |
[MoOCl2(C4H8O)]+ |
255.89 |
256.19 |
8% |
|
[MoOCl2(C4H8O)(C4H6N2)]+ |
337.94 |
338.39 |
1% |
|
[C4H6N2]+ |
82.05 |
83.06 |
100% |
Conclusion
There is an increase in υ(C=N) and torsion and decrease in ring δ(C-H) due to Mo(dπ)→N(pπ) in [1]. 976 cm-1 peak reveals that Mo=O is in terminal position. υ(Mo=O) decreases on N→Mo coordination. Mo=O and the coordinate bond are trans to each other. Molybdenum abstracts oxygen from THF to form Mo=O. Presence of N→Mo coordinate bond is further supported by the 1H NMR in which all absorptions move to lower field, due to flow of π-electron density towards molybdenum. [1] is very much active against microbes. Ions detected in LC-MS suggest the formula presented.
υ(C=N) and torsion show upward shift and ring δ(C-H) shows downward shift owing to Mo(dπ)→N(pπ) [2]. Mo=O is a terminal group is inferred by in the observation of 975 cm-1 peak. N→Mo coordination leads to decrease of υ(Mo=O). Coordinate bond and Mo=O are trans to each other. Molybdenum abstracts oxygen from THF forming Mo=O. N→Mo coordinate bond is further supported by the fact that all 1H NMR absorptions show deshielding, because of depletion of ring π-electron density. Deshielding is further supported by 13C NMR in which absorptions move downfield. Compound is very much potent against microbes. LC-MS fragments noted are in tune with the formula suggested.
υ(C=N) shows an upward shift by 36 cm-1 and torsion shows an upward shift by 24 cm-1 in [3] metal to ligand back bonding. υ(Mo=O) at 980 cm-1 due to refers to Mo=O group in terminal position. υ(Mo=O) declines on coordinate bond formation. Mo=O and the coordinate bond are trans to each other. Molybdenum abstracts oxygen from THF leading to formation of Mo=O. N→Mo coordination is further evident from 1H NMR which shows that all the protons have deshielded on account of decrease in ring π-electron density. Compound can control microorganisms. Fragments observed in mass spectrum conform to the formula.
υ(C=C)shows an increase of 36 cm-1 and υ(N-C) also shows an increase of 39 cm-1 in [4]. This increase is due to inductive effect on coordination of ligand with molybdenum cation and dπ-pπ interactions. Increase in electron density increases the coordinate bond strength. υ(Mo=O) is observed at 978 cm-1 indicating Mo=O being terminal one. N→Mo coordination is further supported by 1H NMR which shows that all the protons have deshielded. Compound has been found to be potent against microbes. Ions observed in LC-MS agree with the formula derived.
Acknowledgement
We are thankful to P. U. Chandigarh, India for extending the instrumental help for characterizing the samples.
Conflict of Interest
There is no conflict of interest among the authors.
References
- Kumar, R. R.; Perumal, S.; Senthilkumar, P.; Yogeeswari, P.; Sriram, D., Bioorg. Med. Chem., 2007, 17, 6459-6462.
CrossRef - Dave, T. K.; Purohit, D. H.; Akbari, J. D.; Joshi, H.S., Indian J. Chem., 2007, 46B, 352-356.
- Ramesh, D.; Chandrashekhar, C.; Vaidya, V. P., Indian J. Chem., 2008, 47B, 753-758.
- Abdel-Latif, N. A.; Sabry, N. M.; Mohamed, A. M.; Abdulla, M. M., Monatshefte Chem., 2007, 138, 715-724.
CrossRef - Subudhi, B. B.; Panda, P. K.; Swain, S. P.; Sarangi, P., Acta Pol. Pharm., 2009, 66(2), 147-153.
- Cocco, M. T.; Congiu, C.; Lilliu, V.; Onnis, V., Bioorg. Med. Chem., 2007, 15, 1859-1867.
CrossRef - Acharya, B. N.; Thavaselvam, D.; Kaushik, M. P., Med. Chem. Res., 2008, 17(8), 487-494.
CrossRef - Amr, A. E. E.; Maigali, S. S.; Abdulla, M. M., Monatshefte fur Chem., 2008, 139, 1409-1415.
CrossRef - Willemann, C.; Grunert, R.; Bednarski, P. J.; Troschutz, R., Bioorg. Med. Chem., 2009, 17(13), 4406-4419.
CrossRef - Singh, T.; Sharma, S.; Srivastava, V. K.; Kumar, A., Indian J. Chem., 2006, 45B, 1557-1563.
- Bahekar, R. H.; Jain, M. R.; Jadav, P. A.; Prajapati, V. M.; Patel, D. N.; Gupta, A. A.;Sharma, A.; Tom, R.; Bandyopadhya, D.; Modi, H.; Patel, P. R., Bioorg. Med. Chem., 2007, 15, 6782-6795.
CrossRef - Bhatia, M. S.; Mulani, A. K.; Choudhari P. B.; Ingale, K. B.; Bhatia, N. M., Int. J. Drug Discov., 2009, 1(1), 1-9.
- Chand, P.; Kotian, P. L.; Morris, P. E.; Bantia, S.; Walsh, D. A.; Babu, Y. S., Bioorg. Med. Chem., 2005, 13(7), 2665-2678.
CrossRef - Buttelmann, B.: Alanine, A.; Bourson, A.; Gill, R.; Heitz, M.; Mutel, V.; Pinard, E.; Trube, G.; Wyler, R., Bioorg. Med. Chem. Lett., 2003, 13(5), 829-832.
CrossRef - Nayak S. G.; Poojary, B., Heliyon, 2019, 5, 1-7.
CrossRef - https://en.wikipedia.org/wiki/4-Methylpyridine.
- https://pubchem.ncbi.nlm.nih.gov/compound/4-Methylpyridine#section=Chemical-Vendors.
- Shimizu, S.; Watanabe, N.; Kataoka, T.; Shoji, T.; Abe, N.; Morishita, S.; Ichimura. H.,Ullmann’s Encyclopedia of Industrial Chemistry, Weinheim: Wiley-VCH, 2007.
- Scriven, E. F. V.; Murugan, R., Kirk-Othmer Encyclopedia of Chemical Technology, Wiley, 2005.
- Iman, M.; Davood, A.; Gebbink, B. K.; Azerang, P.; Alibolandi, M.; Sardari, S., Pharmaceutical Chemistry Journal, 2014, 48(8), 513-519.
CrossRef - Zhu, J.; Lu, J.; Zhou, Y.; Li, Y.; Cheng, J., Zheng, C., Bioorg. Med. Chem. Lett., 2006, 16, 5285-5289.
CrossRef - Weinberg, E. D., Burger’s Medicinal Chemistry and Drug Discovery, J. Wiley & Sons, New York, 1996, 637-652.
- Katritzky, A. R.; Rees, Comprehensive Heterocyclic Chemistry, 1984, 5, 469-498.
CrossRef - Grimmett, M. R., Imidazole and Benzimidazole Synthesis, Academic Press, 1997.
- Brown, E. G., Ring Nitrogen and Key Biomolecules, Kluwer Academic Press, 1998.
CrossRef - Pozharskii, A. F., Soldatenkov, A. T.; Katritzky, A. R., Heterocycles in Life and Society, John Wiley & Sons, 1997.
- Gilchrist, T. L., Heterocyclic Chemistry, the Bath Press, 1985, ISBN 0-582-01421-2.
- Congiu, C.; Cocco, M. T.; Onnis, V., Bioorganic & Medicinal Chemistry Letters, 2008, 18, 989–993.
- Venkatesan, A. M.; Agarwal, A.; Abe, T.; Ushirogochi, H. O.; Santos, D.; Li, Z.; Francisco, G.; Lin, Y. I.; Peterson, P. J.; Yang, Y.; Weiss, W. J.; Shales, D. M.; Mansour, T. S., Bioorg. Med. Chem., 2008, 16, 1890-1902.
CrossRef - Nakamura, T.; Kakinuma, H.; Umemiya, H.; Amada, H.; Miyata, N.; Taniguchi, K.; Bando, K.; Sato, M., Bioorganic & Medicinal Chemistry Letters, 2004, 14, 333-336.
CrossRef - Han, M. S.; Kim, D. H., Bioorganic & Medicinal Chemistry Letters, 2001, 11, 1425-1427.
CrossRef - Roman, G.; Riley, J. G.; Vlahakis, J. Z.; Kinobe, R. T.; Brien, J. F.; Nakatsu, K.; Szarek,W. A., Bioorg. Med. Chem., 2007, 15, 3225-3234.
CrossRef - Bbizhayev, M. A., Life Sci., 2006, 78, 2343-2357.
CrossRef - Nantermet, P. G.; Barrow, J. C.; Lindsley, S. R.; Young, M.; Mao, S.; Carroll, S.; Bailey, C.; Bosserman, M.; Colussi, D.; McMasters, D. R.; Vacca, J. P.; Selnick, H. G., Bioorg. Med. Chem. Lett., 2004, 14, 2141-2145.
CrossRef - Adams, J. L.; Boehm, J. C.; Gallagher, T. F.; Kassis, S.; Webb, E. F.; Hall, R.; Sorenson, M.; Garigipati, R.; Griswold, D. E.; Lee,J. C., Bioorg.Med. Chem.Lett., 2001, 11, 2867-2870.
CrossRef - Thomas, D. D.; Ridnour, L. A.; Isenberg, J. S.; Flores, S. W.; Switzer, C. H.; Donzelli, S.; Hussain, P.; Vecoli, C.; Paolocci, N.; Ambs, S.; Colton, C. A.; Harris, C. C.; Roberts, D. D.; Wink, D. A., Free Radical Biology and Medicine, 2008, 45(1), 18-31.
CrossRef - Chen, P. R.; He, C., Current Opinion in Chemical Biology, 2008, 12(2), 214-21.
CrossRef - Pennella, M. A.; Giedroc, D. P., Biometals, 2005, 18(4), 413-28.
CrossRef - Cowan, J. A.; Bertini, I.; Gray, H. B.; Stiefel, E. I.; Valentine, J. S., Structure and Reactivity: Biological Inorganic Chemistry, 3, University Science Books, Sausalito, 2007, 8(2), 175181.
- Jameel, A.; MSA, S. A. P., Asian Journal of Chemistry, 2010, 22(12), 3422-48.
- Anupama, B.; Sunuta, M.; Leela, D. S.; Ushaiah; Kumari, C. G., Journal of Fluorescence, 2014, 24(4), 1067-76.
CrossRef - Singh, G.; Mangla, V.; Goyal, M.; Singla, K.; Rani, D., American International Journal of Research in Science, Technology, Engineering & Mathematics, 2014, 8(2), 131-136.
- Singh, G.; Mangla, V.; Goyal, M.; Singla, K.; Rani, D., American International Journal of Research in Science, Technology, Engineering & Mathematics, 2015,10(4), 299-308.
- Singh, G.; Mangla, V.; Goyal, M.; Singla, K.; Rani, D., American International Journal of Research in Science, Technology, Engineering & Mathematics, 2015,11(2), 158-166.
- Singh, G.; Mangla, V.; Goyal, M.; Singla, K.; Rani, D.; Kumar, R., American International Journal of Research in Science, Technology, Engineering & Mathematics, 2016, 16(1), 56-64.
- Singh, G.; Kumar, R., American International Journal of Research in Science, Technology, Engineering & Mathematics, 2018, 22(1), 01-08.
- Rani, D.; Singh, G.; Sharma, S., Oriental Journal of Chemistry, 2020, 36(6), 1096-1102.
CrossRef - Rani, D.; Singh, G.; Sharma, S., Oriental Journal of Chemistry, 2021, 37(1), 46-52.
CrossRef - Rani, D.; Singh, G.; Sharma, S., Oriental Journal of Chemistry, 2021, 37(2), 459-466.
CrossRef - Kumar, R.; Singh, G., Oriental Journal of Chemistry, 2021, 37(3), 634-642.
CrossRef - Kumar, R.; Singh, G., Oriental Journal of Chemistry, 2021, 37(3), 553-567.
CrossRef - Vogel, A. I., A Text Book of Quantitative Inorganic Analysis, John Wiley and Sons, New York (Standard methods), 1963.
- Abod, N. A.; M. AL-Askari; Saed, B. A., Basrah Journal of Science (C), 2012, 30(1), 119-131.
- Mohan, J., Organic Spectroscopy: Principles and Applications, CRC Press, 2004.
- Barraclough, C. G.; Kew, D. J., Australian J. Chem., 1970, 23, 2387-2396.
CrossRef - Ward, B. G.; Stafford, F. E., Inorg. Chem., 1968, 7, 2569.
CrossRef - Toco’n, I. L.; Woolley, M. S.; Otero, J. C.; Marcos, J. I., Journal of Molecular Structure, 1998, 470, 241-246.
CrossRef - Gupta, S. K.; Srivastava, T. S., J. Inorganic and Nuclear Chem., 1970, 32, 1611-1615.
CrossRef - Hossain, A. G. M. M.; Ogura, K., Indian J. Chem., 1996, 35A, 373-378.
- Brewerp, D. G.; Wong, P. T. T.; Sears, M. C., Canadian J. Chem., 1968, 46(20), 3119-3128.
CrossRef - Stamboliyska, B. A.; Binev, Y. I.; Radomirska, V. B.; Tsenov, J. A.; Juchnovskiet, I. N., Journal of Molecular Structure, 2000, 516, 237-245.
CrossRef - Uno, T.; Machida, K., Bulletin of the Chemical Society of Japan, 1962, 35(2),276-283.
CrossRef - Amah, K. U.; Sylvain, A. Y. G.; Gaston, K. A.; Alice, K. H. M. T.; Baptiste, M. J., International Research Journal of Pure & Applied Chemistry, 2016, 12(4), 1-11.
CrossRef - Planinic, P.; Meider, H.; Yeh, H.; Vikic-Topic, D., J. Coord. Chem., 1992, 25, 193-204.
CrossRef - Behzadi, K.; Baghlaf, A. O.; Thompson, A., J. Less Common Metals, 1978, 57, 103-110.
CrossRef - Arici K; Gul, O., International J. Chemistry and Technology., 2018, 2(2), 141-152,
CrossRef - Mangla, V.; Singh, G., Oriental Journal of Chemistry, 2019, 35(3), 1094-1102.
CrossRef - Van K. C. G.; Reedijk, J., Inorganica Chimica Acta., 1978, 30, 171-177.
CrossRef - Gottlieb, H. E.: Kotlyar, V.; Nudelman, A., J. Org. Chem., 1997, 62, 7512-7515.
CrossRef - Kumari, N.; Sharma, M.; Das,, P.; Dutta, D. K., Applied Organomet. Chem., 2002, 16, 258-264.
CrossRef - Zamani, K.; Khaledi, M.; Foroughifar, N.; Mahdavi, V., Turk. J. Chem., 2003, 27, 71-75.
CrossRef - https://www.chemicalbook.com/SpectrumEN_108-89-4_13CNMR.htm.
- Bhattacharjee, M. K., The Journal of Antibiotics, 2015, 68, 657-659.
CrossRef - Audi, G.; Wapstra, A. H., Nucl. Phys. A. 1995, 595, 409-480.
CrossRef
This work is licensed under a Creative Commons Attribution 4.0 International License.