Secondary Metabolites Screening, in Vitro Antioxidant and Antidiabetic Activity of Marine Red Alga Botryocladia Leptopoda (J.Agardh) Kylin
Suthan P and Selva Maleeswaran p
Department of Biotechnology, Muthayammal College of Arts and Science, Rasipuram, Tamil Nadu, India.
Corresponding Author E-mail: maleeswaran83@gmail.com
DOI : http://dx.doi.org/10.13005/ojc/380102
Article Received on : 05-Jan-2022
Article Accepted on : 01-01-2022
Article Published : 10 Jan 2022
Reviewed by: Dr. Shaiphali Saxena
Second Review by: Dr. Suthan P
Final Approval by: Dr. Tanay Pramanik
Today, seaweed has become essential to medicine due to its ability to treat disease-afflicted cells without majorly influencing healthy cells and tissues. This study included a phytochemical analysis, in vitro antioxidant and antidiabetic activity of all the extracts of Botryocladia leptopoda. Also, analyse the cytotoxicity and glucose absorption activity of the ethanolic extract of B. leptopoda using 3T3 cell line through MTT assay. The extraction of secondary metabolites from B. leptopoda using various solvents (hexane, chloroform, ethyl acetate, ethanol and water) resulted in alkaloids, Phenol and Flavonoids. The ethanolic extract of B. leptopoda showed the best in vitro antioxidant and antidiabetic activity compared with all the experimented extracts. Ethanolic extract exhibited no cytotoxicity and improved glucose absorption. This study has revealed that marine red alga B. leptopoda showed noticeable in vitro antioxidant, antidiabetic activities and no toxic effect toward normal cells. We suggested to carry out the antidiabetic activity through in vivo investigations on Botryocladia leptopoda (J.Agardh) Kylin.
KEYWORDS:Botryocladia Leptopoda; In Vitro Antioxidants; In Vitro Antidiabetic Study; Marine Red Alga
Download this article as:
Copy the following to cite this article: Suthan P, Maleeswaran P. S. Secondary Metabolites Screening, in Vitro Antioxidant and Antidiabetic Activity of Marine Red Alga Botryocladia Leptopoda (J.Agardh) Kylin.Orient J Chem 2022;38(1). |
Copy the following to cite this URL: Suthan P, Maleeswaran P. S. Secondary Metabolites Screening, in Vitro Antioxidant and Antidiabetic Activity of Marine Red Alga Botryocladia Leptopoda (J.Agardh) Kylin.Orient J Chem 2022;38(1).Available from: https://bit.ly/34CEFaX |
Introduction
The sea provides an abundance of beneficial seaweeds with biodynamic properties. More people are interested in the secondary metabolites polyphenols, carotenoids, terpenes, and alkaloids due to their antioxidant capabilities and positive impact on the human body1. Many studies have been done on plants to discover their bioactive compounds, but this branch of investigation has much less been pursued in marine algae. The technique will naturally use organic solvents, such as hexane, chloroform, ethyl acetate, ethanol and water, since many of these extraction processes have been done in those solvents2. An additional benefit of using alcoholic extracts is that they facilitate the extraction of polyphenols3. Algae has long been used as a food resource for animals and humans alike. Proteins, carbohydrates, lipids, minerals, vitamins, and bioactive substances, such as polyphenols and carotenoids, are abundant in macroalgae. Marine algae and seagrass primarily produce the latter compounds to protect their structural and ecological purposes. Yet, their crude materials, like antioxidants, anti-inflammatory, antidiabetic, antibacterials, antivirals, and other disease-preventive agents, have interesting properties4. The health benefits of marine macroalgae are primarily due to their capacity to lower oxidative stress through their radical-scavenging capabilities. The marketing of algae is centred on their usage as nutrients and dietary supplements, generating nontoxic, antioxidative functional products.
Marine algae contain significant pharmacological and biologically active components such as carotenoids, flavonoids, terpenoids, dietary fibre, essential fatty acids, protein, minerals, and vitamins. The attention in marine algae as a potential source of capable new bioactive natural agents has expanded late. The secondary metabolites from marine red algae are widely used in all kinds of ventures5. Marine red algae have well-known abilities to fight diseases, infections, and illnesses thanks to their extensive and effective bioactivities, including anti-inflammatory, antipyretic, analgesic, and antiparasitic properties6. Marine red algae have been almost inextricably linked to human life.
They have been extensively employed as a source of nourishment, feed, fertiliser, and medicine throughout history and are mainly used for economically relevant phycocolloids. Many of India’s rocky shorelines, mudflats, estuaries, coral reefs, and tidal ponds provide ideal environments for seaweed growth. In total, 271 genera and 1153 species of seaweed have been recognized in Indian waters to date. Rhodophyta is commonly referred to as ‘the red alga,’ and the majority are tiny7. The phytochemical analysis, free radical scavenging, and antidiabetic activity of these algae have been largely unexplored, and many elements of their biological potential remain unknown. The intention of this study is to determine the phytochemical content, in vitro free radicle scavenging activities and antidiabetic potential of Botryocladia leptopoda (J. Agardh) Kylin (Family: Rhodymeniaceae) species collected in Mandapam, Tamil Nadu, India.
Materials and Methods
Seaweed collection
Botryocladia leptopoda (J.Agardh) Kylin was obtained in September-December 2017 from the rocky intertidal beach of Mandapam, Gulf of Mannar, Tamil Nadu, India. In order to eliminate the salt, bacteria, and other sediment that was present in the water, fresh samples were first rinsed with saltwater but then with clean water. After the samples were allowed to dry for two weeks, they were put away at room temperature..
Preparation of extracts
The dried in the shade, B. leptopoda sample was coarsely powdered, and the powder was stored in sterile containers for subsequent processing. The coarsely powdered material was extracted with hexane (HeBl), chloroform (ChBl), ethyl acetate (EaBl), ethanol (EtBl), and water (AqBl). To dry the extract, a spinning vacuum evaporator was used, with a vacuum pump and cryostat connected. The crude extracts produced were used to conduct more research.
Phytochemical Analysis
The presence and absence of secondary metabolites such as steroids, Glycosides, Anthraquinones, Phenol, Alkaloids, Triterpenoids, Tannin, Saponin, and Flavonoids were determined using qualitative chemical assays utilizing different solvents (Hexane, Chloroform, Ethyl acetate, Ethanol, and water)8.
Determination of phytoconstituents
Estimation of Total Alkaloids
To isolate an aqueous layer with acidic properties, a solution of 0.1N HCl was added to the algal sample. Then the algal sample was partitioned using a separating funnel with chloroform. To prepare the chloroform layer for the extraction, it was removed, and the aqueous layer was adjusted to an alkaline pH using ammonium hydroxide. A separating funnel was used to partition the aqueous and chloroform layers. To isolate the alkaloids, the chloroform was evaporated, and the water layer was removed. The total alkaloid content was treated as such, and its existence was confirmed with Dragendroff’s reagent9.
Estimation of Total Flavonoids
Ethyl acetate was used to extract the aqueous algal extract. The extracts have been dried, filtered and vacuum-concentrated with anhydrous sodium sulphate to 1g/ml extract. They then make a 0.01g/ml solution using ethyl acetate to be used in the tests. 1ml of 2% aluminium chloride was put to a 25ml volumetric flask, then filled with methanol-acetic acid and 10ml of the original solution. After thirty minutes, the absorbance was measured at 390 nm. Additionally, a blank was preserved. The calibration curve was created using luteolin at values ranging from 1 to 10g/ml10
Estimation of Phenol
weighed and thoroughly ground 0.5 to 1g of the material with 10 times the volume of 80% ethanol. The homogenate was spun at 10,000rpm for 20 minutes. After the supernatant was removed, the remaining extract was re-extracted five times with 80% ethanol. The supernatant was removed using a centrifuge. It was then desiccated.. 5ml of distilled water was added to the residue obtained. After you fill up the pipette with 0.2-2ml samples, transfer them to test tubes. The solution was made 3ml more concentrated by adding water.. After 3 minutes, all tubes were combined with 0.5ml of Folin’s reagent and 2ml of 20% sodium bicarbonate solution. The contents were thoroughly mixed, and the test tubes were immersed in boiling water for 1 minute, cooled, and the colour generated at 650nm was measured11.
In vitro Antioxidant activity
DPPH radical scavenging assay
The DPPH radical scavenging assay results in a colour change from purple to yellow when exposed to hydrogen donors. For testing, the antioxidant was mixed with DPPH and standardised with ascorbic acid. To 1ml of algal extracts of various strengths, 0.2mg of DPPH solution in methanol was added, and the mixture was shaken for 24 hours to give a 4.0ml solution (0.2mg in methanol). The absorbance was measured at 517nm after incubation for 30 minutes at room temperature12.
ABTS Radical Scavenging Assay
5ml of a 4.9mM potassium persulphate solution was added to 5ml of a 14mM ABTS solution to make a fresh ABTS solution. The resulting solution was kept at room temperature (251°C) in the dark for 16 hours. This solution was diluted with methanol to obtain an absorbance at 734nm for the antioxidant assay, and the exact resolution was used. 1ml of the reaction mixture containing standard and extracts had 950L ABTS solution and 50L samples. After 10 seconds of vortexing, the absorbance at 734nm was measured using a UV-visible spectrophotometer compared to the control ABTS solution13.
In Vitro α-amylase and α-glucosidase Inhibitory Assay
α-Amylase Inhibitory Activity
Incubation was conducted at 25°C for 10 minutes using 500μl of HeBl, ChBl, EaBl, EtBl, and AqBl and the standard drug (25-1000 µg/ml) in 0.02M phosphate buffer (pH 6.9) and contained α-amylase (0.5 mg/ml). 0.02 M sodium phosphate buffer (pH 6.9) was added to each tube, which contained 500μl of 1% starch solution. After being incubated at 25°C for 10 minutes, the reaction mixtures were allowed to stand. The reaction was quenched with 1.0ml of 3, 5-dinitrobenzene-sulfonic acid (DNS) indicator solution. The test tubes were then allowed to cool down to room temperature after being incubated in a boiling water bath for 5 minutes. After the solution was diluted, the absorbance was read at 540nm.14.
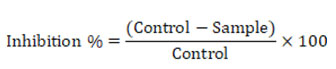
Alpha-Glucosidase Inhibitory Activity
The procedure of Matsui et al.15 was followed; however, several adjustments were made. Algal sample and acarbose (25, 50, 75, and 100 µg/mL) concentrations were tested in acetic buffer at 37ºC for 5 minutes, with or without PNPG solution. A 25mU/mL stock solution of α-glucosidase was prepared and incubated at 37°C for 35 minutes. NaCO3 solution was used to halt the reaction after incubation. Then, the absorbance was measured at 400nm. The control sample was utilised to measure the anti-glucosidase activity in this experiment.
Glucose Diffusion Inhibitory Activity
This method of in vitro testing uses algal extracts to examine the influence on glucose transport. A dialysis bag was sealed at each end with 1ml of 0.15M sodium chloride containing 0.22M D-glucose injected to it. For the experiment, 1ml of 2.5g/100 ml extract and 1 ml of 0.15 M sodium chloride with 0.22 M D-glucose were combined in a dialysis bag16,17. The dialysis bag was maintained at normal temperature using a magnetic stirrer. The glucose content of external 0.15 M NaCl was ascertained through using dinitrosalicylic acid method, with the results showing an increase in glucose concentration after 1, 3, 6, 12, and 24 hours of incubation. 1ml of the sample was placed to 1ml of dinitrosalicylic acid, and the mixture was heated for 15 minutes. The absorption at 540 nm was then measured18.
Determination of cell viability by MTT assay
To modify the cell count, trypsinise the monolayer cell culture, then divide it into two 1.0 x 105 cells/ml fractions using DMEM with 10% FBS. 0.1ml of the diluted cell suspension (around 10,000 cells) was added to each 96 well microtitre platform. A microtitre plate was created using a partial monolayer by leaving it for 24 hours after the medium was completely absorbed. The supernatant was removed, and the monolayer was washed with medium once before 100µl of test drug concentrations were added. The plates were then incubated in a 5% CO2 atmosphere at 37ºC for three days. For the study, a microscope was used, and records were taken daily. The well-solutions were withdrawn after three days, at which point each well was incubated with 50µl of MTT in PBS. After gently shaking the plates, they were incubated for 3hours at 37oC in a 5% CO2 environment. 100μl of propanol was poured, and the plates were gently shaken to dissolve the generated formazan. The absorbance was ascertained using a microplate reader at a wavelength of 540nm. This formula was used to determine the percentage growth inhibition. The CTC50 values were obtained using each cell line’s dose-response curves19.

In vitro glucose uptake assay
The absorption of glucose by test medicines was measured in the differentiated 3T3 cell line. The differentiated cells were obtained in 40mm Petri dishes with a 70-80% confluency and were kept in DMEM with 2% FBS for 4-6 days. The levels of cell proliferation were determined by examining multinucleation. The serum-starved differentiated cells were left overnight. Following the experiment, the cells were washed with a buffer solution of HEPES and Krebs Ringer Phosphate (KRP) once, then incubated with a solution of KRP and 0.1% BSA for 30 minutes at 37°C. Two distinct nontoxic concentrations of the test and standard medicines were applied to the cells for 30 minutes and controls at 37°C. Each well was injected with D-glucose solution and incubated at 370°C for 30 minutes. The growth of glucose absorption was stopped by removing the fluid from the wells and giving three washes with ice-cold KRP buffer. A sample of lysed cells was utilised to quantify the amount of glucose connected with the cells. A glucose tolerance test kit was used to check glucose levels in cell lysates. To quantify the glucose intake of each test group, three independent duplicates were used20.
Statistical analysis
All of the tests were done in triplicate, and MS-Excel was used to calculate the means and standard error of the mean (SEM). The results were presented as mean±standard error of the mean (SEM).
Results and Discussion
Qualitative Phytochemical screening
The medico-pharmaceutical industry makes considerable use of active metabolites, which are highly useful to both people and animals. As a result, the current study of phytochemical analysis may aid producers in discovering and accumulating raw resources for the manufacture of pharmaceuticals. Seaweed is a natural nutraceutical because of these biological characteristics. They are crucial as dietary supplements in this approach, with the ultimate goal of enhancing general well-being and treating disease.
The phytochemical constituents results in Table 1 indicated the presence or absence of specific phytochemicals in the drug, highlighting the critical need to standardise crude pharmaceuticals. It has become crucial for the identification and authentication of pharmaceutical products. Flavones, alkaloids, and phenols were detected in all B. leptopoda preparations used in this investigation. Except for hexane, all examined sections contained anthraquinones, triterpenoids, and saponin. Steroid, glycoside, and tannin were detected in chloroform, ethanol, and water extracts. The phytochemical study findings may imply that alga may be beneficial in treating various diseases and may even provide helpful pharmaceuticals for human use. Further research is necessary to ascertain the pharmacological activity of B. leptopoda extracts.
Table 1: Preliminary phytochemical investigation of different extracts of B. leptopoda
S. NO. |
Phytochemicals |
Extraction of different solvents |
||||
HeBl |
ChBl |
EaBl |
EtBl |
AqBl |
||
1. |
Steroids |
– |
+ |
– |
+ |
+ |
2. |
Glycosides |
– |
+ |
– |
+ |
+ |
3. |
Antheraquinones |
– |
+ |
+ |
+ |
+ |
4. |
Phenol |
+ |
+ |
+ |
+ |
+ |
5. |
Alkaloids |
+ |
+ |
+ |
+ |
+ |
6. |
Triterpenoids |
– |
+ |
+ |
+ |
+ |
7. |
Tannin |
– |
+ |
– |
+ |
+ |
8. |
Saponin |
– |
+ |
+ |
+ |
+ |
9. |
Flavonoids |
+ |
+ |
+ |
+ |
+ |
Seaweeds are beneficial in treating various ailments and have the potential to produce valuable pharmaceuticals for human use due to their phytoconstituents. These phytoconstituents exhibit antibacterial and antifungal21, antiviral22, anticoagulant23, anticancer24, and anti-inflammatory25 properties.
The two metabolic pathways for algal steroids are a diverse group of compounds that can be synthesised. Conditions such as delayed puberty, cancer and AIDS-related muscle loss can be treated with steroids. The adrenal glands benefit from steroids, which help treat illness and injury-related stress. They help fight inflammation and bolster the immune system. Steroids are used to assist in treating various medical conditions in which the body’s defence mechanism is not working correctly, causing damage to the tissue26.
When a sugar’s anomeric carbon hydroxyl group joins with the hydroxyl group of another particle to generate an acetal or metal connection, that’s referred to as a glycosidic bond. The presence of glycosides may aid the identification of antioxidant and anti-inflammatory effects in the investigated alga. It is also possible that this alga may be used as a supplement in the treatment of cancer. The macroalgae Zostera sp., Zhongguo Haiyang Yaowu, and H. porphyroides, Yang et al. (1992) discovered, contain glycosides27. Glycosides were present in lower concentrations in these algae than in B. leptopoda.
The volatile chemical compound anthraquinone is aromatic and has a pungent smell. It is quinones utilised to treat malaria and cancer. They could be anti-inflammatory, antimicrobial, and immunomodulatory agents. To combat the substance’s severe flammability, the use of anthraquinone in the synthesis of different compounds safeguards the majority of producers. Furthermore, B. leptopoda extracts showed anthraquinones in the methanol extracts28.
In terms of the human body, phenols are known to play a more significant part in maintenance29. Seaweed extracts are full of phenolic compounds, according to a recent study. Phenolic compounds are a common antioxidant in brown alga30. When phenolic compounds, which are the chemical components of algal cells, are produced and concentrated, they may affect the action of microbes in an activating or inhibiting way. Furthermore, seaweeds have been demonstrated to inhibit oxidative phosphorylation, as well as causing lysis of bacterial proteins, such as enzymes and cell membrane proteins, by their capacity to attach to them31. The biological and pharmacological properties of phenolic compounds include antibacterial, antiviral, anti-inflammatory, cytotoxic and anticarcinogenic.
Alkaloids are complex nitrogen molecules found in plants, microbes, animals, and marine creatures and are naturally occurring. The alkaloids in marine algae are scarce compared to those in terrestrial plants, and their applications are unclear. Most indole alkaloids are found in Rhodophyta32. A wide range of pharmacological effects, including neuronal regulation, neurotransmission, growth control, cytotoxicity, angiogenesis, and antioxidant activity, are exhibited by a number of these alkaloids, in addition to antibacterial, antifungal, and larvicidal properties. Macroalgae produce a diverse range of bisindole alkaloids with exciting bioactivities, which have caused a great deal of excitement in the scientific community33.
Biogenic compounds called triterpenoids are metabolite activators. The Tolypiocladia glomerulata red alga was less prevalent as compared to the marine algae. Studies have shown that there are several triterpenes in seaweed34. The diterpenes and meroditerpenes produced by red seaweeds and Ghazala are among macroalgae’s most commonly used and economically essential terpenoids35.
Tannins are polyphenols that are present both in marine and terrestrial plants. Tannins, at low concentrations, act as an antifungal agent by coagulating the microorganism’s protoplasm, while higher amounts hinder the growth of microorganisms36. Halymenia poryphyroides37 had the lowest tannin concentration, whereas B. leptopoda had the highest. S. polycystum and T. ornata extracts contain tannins, polyphenols, and cardiac glycosides, according to research38.
Traditional medicine, which is based on Saponins, is widely used, and Saponins are the cause of the bulk of the biological effects experienced. Saponins have many different biological actions, including antibacterial, anti-inflammatory, and antifeedant properties and hemolytic capabilities. Saponins have a wide range of biological effects, including hemolyse (break down blood cells) and serve as an antibacterial agent. Saponins are utilised to treat hypercholesterolemia, hyperglycemia and as an anti-inflammatory, anticancer agent, antioxidant substance, and weight-loss aid. Saponins are also beneficial in treating cancers, and studies have shown that they can effectively target and inhibit tumour angiogenesis by stopping endothelial cells in blood arteries from inducing the development of tumours and limiting the spread of tumour cells39.
Since flavonoids have so many diverse biological and pharmacological effects, they are considered nature’s gentle medication. Research into flavonoid-rich plants has been driven by discoveries in the field of flavonoid characteristics. A recent study discovered that B. leptopoda, a sea-dwelling red alga, has a higher concentration of flavonoids. Flavonoids, as antioxidants, may help lower the prevalence of type 2 diabetes by maintaining the dynamic impedance of pancreatic beta cells injured by oxidative stress40.
Quantitative Analysis of Secondary Metabolites
After successive extractions of B. leptopoda, HeBl, ChBl, EaBl, EtBl, and AqBl were obtained. Quantification of phytoconstituents found in all extracts, including total alkaloid, total phenol, and total flavonoid.
Table 2: Quantitative analysis of important organic constituent
S. No |
Extracts |
Organic Constituents |
||
Alkaloids (mg/g) |
Flavonoids (mg/g) |
Phenols (mg/g) |
||
1 |
HeBl |
1.78±0.29 |
2.05±0.27 |
2.83±0.2 |
2 |
ChBl |
2.99±0.09 |
3.74±0.26 |
3.73±0.19 |
3 |
EaBl |
3.52±0.12 |
4.34±0.31 |
4.34±0.1 |
4 |
EtBl |
4.83±0.60 |
5.06±0.26 |
6.58±0.68 |
5 |
AqBl |
3.97±0.67 |
4.74±0.19 |
4.87±0.33 |
Identifying crude medicines requires the presence of secondary metabolites in Alga. Finding medically and technologically relevant molecules such as flavonoids, alkaloids, and phenolic compounds involves assessing phytochemicals. Table 2 is a summary of the essential organic compounds’ findings in quantitative analysis.
Alkaloids are more diverse, plant-based chemical compounds with many different structures, origins, and functions. These results suggest that alkaloids are present in quantities of 1.78±0.29, 2.99±0.09, 3.52±0.12, 4.83±0.60 and 3.97±0.67mg/g, respectively, in HeBl, ChBl, EaBl, EtBl, and AqBl. These results suggest that EtBl has a high alkaloid content and may have anti-free radical, hepatoprotective and antidiabetic potentials.
Polyphenolic chemicals recognised for their antioxidants, enzyme-inhibiting, and anti-inflammatory activities, flavonoids are a category of compounds. In addition to flavonoids’ antioxidant abilities, their potential to scavenge superoxide anion radicals, hydroxyl radicals, and lipid peroxy radicals highlight several of the organism’s health-promoting benefits. These include protecting the organism’s membrane, proteins, and DNA from oxidative damage, which is crucial in preventing diseases resulting from oxidative damage41. The highest total flavonoid content was found in HeBl (2.05±0.27mg/g), ChBl (3.74±0.26mg/g), EaBl (4.34±0.31mg/g), EtBl (5.06±0.26mg/g), and AqBl (4.74±0.19mg/g). To stave off harm to human cells, flavonoids are a must. Many epidemiological studies have demonstrated a strong correlation between high consumption of flavonoids and lower cardiovascular disease and cancer risks. A surge of literature has been created questioning the well-known significance of flavonoids as antioxidants and cancer fighters. A prior study found that flavonoids may reduce inflammation, oxidative stress, and diabetes symptoms, and they have been used to treat diabetes as a chemopreventive measure42.
Phenols are naturally occurring plant chemicals. They are filled with several active components that help prevent mutations, cancer, and oxidative damage and have the capacity to impact gene expression43. HeBl, ChBl, EaBl, EtBl, and AqBl extracts had a total phenolic content of 2.83±0.20, 3.73±0.19, 4.34±0.10, 6.58±0.68, and 4.87±0.33mg/g, respectively. Furthermore, phenolics can scavenge free radicals, functioning as antioxidants. Phenolics have been discovered to be important in combating cancer, heart disease, and other age-related illnesses. Phenolic compounds in a high quantity help it fight free radicals. The phytoconstituents of B. leptopoda were quantitatively examined. It was discovered that the ethanol extract might be used as a source of medications that scavenge free radicals and treat and reduce a range of ailments44.
Antioxidant assays
We conducted the DPPH and ABTS assays to measure the antioxidant capacity of HeBl, ChBl, EaBl, EtBl, and AqBl. To gauge the antioxidant capabilities of alga, many people rely on the DPPH radical scavenging property45. In vitro tests using a sample of B. leptopoda at 25, 50, 75, and 100mg/ml, and each extract’s DPPH radical scavenging ability was tested. The DPPH radical might be reduced to the positively yellow diphenyl-picrylhydrazine by antioxidants in the DPPH experiment. The process starts with using an antioxidant that supplies hydrogen, causing the DPPH solution to become less concentrated and, as a result, react to generate DPPH-H46. The scavenging capacity of B. leptopoda extracts, shown in Figure 1, is concentration-dependent and allows for an efficient increase in the DPPH radical suppression. The study found that the marine algal ethanol extract has the greatest free radical scavenging activity compared to DPPH, as evidenced by the decrease in DPPH concentration. The inhibitory activity of EtBl compared with Ascorbic acid. The results of the extracts of B. leptopoda reveal that EtBl with high total phenolic content shows considerable radical scavenging activity, which can be related to the inherent nature of phenolic compounds, which contribute to the transfer of electrons or hydrogen capacity. Also, it has been found that certain chemicals with differing polarity have variable antioxidant capabilities, and these capabilities are depending on the solvent utilised. The antioxidant scavenging activities on Pterocladiella capillacea and Osmundaria obtusiloba47, Gracilaria edulis48, Jania rubens49, Rhodomela confervoides50, Actinotrichia fragilis51, and Portieria hornemannii52 also supported this results of EtBl.
![]() |
Figure 1: DPPH radical scavenging activity of B. leptopoda |
ABTS free radical scavenging
This extract was very effective at scavenging ABTS radicals because of the ABTS radicals’ scavenging capabilities. Gracilaria edulis48, Jania rubens49, Rhodomela confervoides50, Actinotrichia fragilis51, and Portieria hornemannii52 all displayed the ability to scavenge ABTS. A radical with an attached proton (ABTS) has a specific absorbance at 734nm that drops with the scavenging of proton radicals53. EtBl, therefore, showed its capability to scavenge ABTS radicals and initiate lipid oxidation by way of a chain-breaking process.
![]() |
Figure 2: Effect of B. leptopoda on the percentage of ABTS activity |
In vitro α-amylase and α-glucosidase Inhibitory Assay
Figure 3 illustrates that crude B. leptopoda extracts exhibited a percentage inhibition of α-amylase activity that increased with increased concentration. The crude extract proved to be the most active fraction in the α-amylase inhibition experiment. The EtBl displayed a percentage inhibition of 21.32, 42.44, 58.84, 75.87, 92.47 and 95.93% at concentrations of 25, 50, 100, 250, 500, and 1000μg/ml, respectively, obtaining IC50 of 95.83μg/ml. The AqBl displayed 17.40, 33.16, 49.41, 68.15, 88.36 and 93.34% inhibitions at the same tested concentrations with an IC50 value of 201.74μg/ml, respectively. The EaBl displayed 16.27, 32.89, 44.75, 63.40, 82.53 and 89.33% inhibitions at the same tested concentrations with an IC50 value of 248.13μg/ml, respectively. Likewise, the ChBl displayed 15.43, 30.28, 44.40, 60.37, 76.77 and 86.73% inhibitions at the same tested concentrations with an IC50 value of 284.85μg/ml, respectively. Similarly, HeBl unveiled 11.28, 22.52, 30.98, 45.21, 63.91, and 75.51% inhibitions at the same tested doses with an IC50 value of 458.76μg/ml, respectively. In comparison, the standard drug acarbose demonstrated 19.52, 32.68, 44.78, 60.54, 79.35, and 93.34% inhibitions at doses of 15.6, 31.3, 62.5, 125.0, 250.0, and 500 μg/ml, respectively, obtaining IC50 of 9.82 μg/ml.
![]() |
Figure 3: Effect of extracts of B. leptopoda against the inhibitory activity of the enzymes α-amylase activity. |
![]() |
Figure 4: Effect of extracts of B. leptopoda against the inhibitory activity of the enzymes α-glucosidase activity. |
Figure 4 shows that B. leptopoda’s crude extracts have α-glucosidase inhibitory action, as demonstrated. The inhibitory effects of the crude extract’s 12.5 to 100 μg/ml concentrations were found to vary depending on the concentration.. As a result, the lowest concentration (12.5 μg/ml) of the crude extract inhibited α-glucosidase by 11.77% (IC50, 12.88 μg/ml), 15.22% (IC50, 19.15 μg/ml), 16.51% (IC50, 21.43 μg/ml), 18.33% (IC50, 24.39 μg/ml), 20.31% (IC50, 27.31 μg/ml), and 21.52% (IC50, 30.24 μg/ml), respectively, for ChBl, HeBl, EaBl, AqBl, EtBl, and acarbose.
Decreased postprandial hyperglycemia occurs when intestinal and pancreatic α-amylase and α-glucosidase activity are inhibited54. In the current investigation, the B. leptopoda crude extract demonstrated α-amylase inhibitory action. It is a striking strategy for managing postprandial hyperglycemia to look for a new α-amylase inhibitor from medicinal plants. The primary phytoconstituents that have α-amylase inhibitory action are tannins, phenolic acids, and flavonoids55. A recent study identified polyphenols with α-amylase inhibitory activity in crude extract and solvent fractions56. The latest research shows that the solvent’s polarity affects the α-amylase inhibitory properties of the extracts (ChBl, HeBl, EaBl, AqBl, and EtBl). It may be hypothesised that the crude extract, which contains more significant quantities of phytoconstituents for α-amylase inhibition, has a greater effect on α-amylase inhibition than the solvent fractions.
Management of Diabetes Mellitus (DM) may be improved with therapeutic inhibition of α-glucosidase, one of the postprandial hyperglycemia treatments (delaying glucose absorption). Antidiabetic medication market share, as measured by the alpha-glucosidase inhibitor class, has been the management of DM57. It is claimed in the literature that the crude extract is rich in phenolic chemicals, which may contribute to its in vitro antidiabetic action58. The recent studies proved that the α-amylase and α-glucosidase inhibitory activity red marine alga-like Bangia fusco-purpurea59, Padina tetrastromatica60, Gracilaria opuntia61 and Ceramium rubrum62. Additionally, the EtBl had the most significant inhibition of α-amylase and α-glucosidase.
Glucose Diffusion Inhibitory Activity
The EtBl extract was shown to be more powerful than other extracts in inhibiting glucose transport, with the least value glucose concentration of 363.33μg/ml in an external medium after 24 hours. The glucose concentration in external solution in EtBl in the decreasing order was AqBl> EaBl> ChBl > HeBl. The mean glucose level in the external medium had plateaued 24 hours after dialysis treatment began, and the average concentration of glucose in the external medium was 390.52 μg/ml, shown in Figure 5.
![]() |
Figure 5: Effect of extracts of B. leptopoda on the movement of glucose diffusion out of dialysis tube |
To assess the effect of EtBl on glucose retardation, the glucose diffusion inhibition test was used. The efficacy of the EtBl and four extracts to trap glucose differed significantly over time. The EtBl, in particular, show a significant ability to entrap glucose, and it was delivered to slow the passage of glucose into the external solution compared to the control at 180 minutes. The existence of insoluble fibre molecules that trap glucose molecules could explain why the ethyl acetate portion inhibited glucose transit the most63. A simple method of determining the effect of EtBl and other extracts to retard glucose diffusion through the normal dialysis membrane, the dialysis tube method relies on the EtBl and other extracts, which, in the intestinal tract, aids glucose transportation, thanks to glucose transporters that are integrated with other molecules and the assistance of intestinal contractions64. It is thus essential to further research the effects of EtBl and other extracts on glucose diffusion in vivo.
Determination of cell viability by MTT assay
Many cytotoxicity experiments have focused on cell survival under harsh conditions. It relies on both the number of cells that can be used and the activity of the mitochondria in cells. The MTT assay is premised on the notion that dead cells or their byproducts do not react with tetrazolium. Metabolically active cells are the only ones capable of reducing tetrazolium salts. The enzyme succinate dehydrogenase converts MTT to a blue formazan, so its levels can be lowered. Formazan production correlates directly with the quantity of live cells65. The MTT test was used to measure the cytotoxicity of the ethanolic extract of B. leptopoda against three cell lines, including 3T3, at varying doses. The IC50 value was then determined. EtBl extract was found to have dose-dependent cytotoxic effects. 3T3 cells are susceptible to the toxicity of different cell lines. Despite being more toxic to cancer cells, the extracts were less likely to kill normal cells66. This investigation showed that the ethanol extract is harmless and therefore can be regarded as nontoxic and safe. This investigation has shown that it is safe to eat. Next, they did testing to see how well the extracts took up glucose. The dosage was decided for glucose uptake utilising cell lines based on cytotoxicity assays.
![]() |
Figure 6: In vitro cytotoxic effect of Botryocladia leptopoda using MTT Assay |
Glucose uptake activity of ethanolic extract of Botryocladia leptopoda using 3T3 cell lines
Skeletal muscle is a significant component that plays a role in insulin-promoted glucose uptake. The plasma membrane in skeletal muscle has glucose transport molecules that promote glucose uptake thanks to insulin. Muscular contractions can also improve skeletal muscle glucose transport. In Type 2 diabetes, defects in insulin-stimulated skeletal muscle glucose absorption are prevalent clinical conditions.
A well-studied cellular model to investigate glucose absorption and GLUT4 translocation in the 3T3 cell line. Based on this investigation, the effect of the ethanolic extract of B. leptopoda on glucose uptake is examined in the 3T3 cell line, as seen in Figure 7 and Table 7. Ethanol extract of B. leptopoda was discovered to be more significant compared to the control in glucose consumption in 3T3 cell lines. Insulin and metformin, which are standard antidiabetic medicines, were utilised to compare these results. Insulin and metformin were discovered to boost glucose absorption when used at concentrations of 1IU/ml and 100 µg/ml, respectively.
![]() |
Figure 7: These photos show the microscopic image of 3T3 cell line morphology after the 24-h incubation period with various concentrations (20 – 100mg/ml) of extracts of EtBl. EtBl did not alter cell morphology at any of the tested concentrations |
Table 3: In vitro glucose uptake studies in 3T3 cell line
S. No. |
Concentration (µg/ml) |
% Glucose uptake over control |
1 |
Glucose induced Control |
0 |
2 |
EtBl – 20 |
19.74 |
3 |
EtBl – 40 µg/ml |
38.74 |
4 |
EtBl – 60 µg/ml |
48.91 |
5 |
EtBl – 80 µg/ml |
75.99 |
6 |
EtBl – 100 µg/ml |
87.36 |
![]() |
Figure 8: These photos show the microscopic image of in vitro glucose uptake studies in 3T3 cell line morphology after the 24-h incubation period with various concentrations (20 – 100mg/ml) of extracts of EtBl. |
B leptopoda is thus considered safe and nontoxic and is also likely to be an effective source of glucose absorption. Adipose tissue contains GLUT-4, a glucose transporter, stored inside a cell before being relocated to the plasma membrane. In the study’s results, B. leptopoda ethanolic extract improved glucose absorption in vitro settings. Phytoconstituents in the B. leptopoda or some other factor could be responsible for this phenomenon. However, it’s necessary to test these results in vivo by performing various in vivo research and clinical trials to evaluate their utility as therapeutic agents.
Conclusion
Marine Red algae have always been beneficial to humanity by treating health problems. The findings from this study were compared to the recently published phytochemical content of seaweeds, which was utilised to determine the presence of alkaloids, tannins, saponins, flavonoids, glycosides, and phenols. The marine genus produces active ingredients that are used in complementary and alternative medicine. Diverse marine alga have been discovered to possess active compounds capable of curing ailments. Nowadays, many people prefer to employ natural therapies to treat illnesses, as these are said to have fewer adverse effects.
Conflict of Interest
There is no conflict of interest
Funding Sources
There is no funding source.
References
- Dillard, C. J.; German, J. B. J. Sci. Food Agric. 2000, 80(12), 1744-1756.
CrossRef - Cotas, J., Leandro, A., Monteiro, P., Pacheco, D., Figueirinha, A., Gonçalves, A. M., Pereira, L. Mar. Drugs. 2020, 18(8), 384.
CrossRef - Mohanty, S., Pradhan, B., Patra, S., Behera, C., Nayak, R., Jena, M. J. Adv. Plant Sci. 2020,10, 1-8.
- Suleria, H. A. R., Osborne, S., Masci, P., Gobe, G. Mar. Drugs. 2015, 13(10), 6336-6351.
CrossRef - Barbalace, M.C., Malaguti, M., Giusti, L., Lucacchini, A., Hrelia, S., Angeloni, C., Int. J. Mol. Sci. 2019, 20(12), 3061.
CrossRef - Rao, P. S., Mantri, V. A. Curr. Sci. 2006, 164-174.
CrossRef - Kunatsa, Y., Chidewe, C., Zvidzai, C.J., J. Sci. Food Agric. 2020, 2, 100027.
CrossRef - Dube, N., van Heerden, F.R., Zachariades, C., Uyi, O.O., Munyai, T.C., S. Afr. J. Bot. 2021, 139, 19-25.
CrossRef - Malik, C. P., Singh, M. B. Kalyani Publishers, New Delhi, India. 1980, 286.
- Gyamfi, M. A., Aniya, Y. Biochem. Pharmacol, 2002, 63(9), 1725-1737.Re, R., Pellegrini, N., Proteggente, A., Pannala, A., Yang, M., Rice-Evans, C. Free Radic. Biol. Med. 1999, 26(9-10), 1231-1237.
CrossRef - Nair, S. S., Kavrekar, V., Mishra, A. Eur. J. Exp. Biol. 2013, 3(1), 128-132.
- Matsui, T., Ueda, T., Oki, T., Sugita, K., Terahara, N., Matsumoto, K. J. Agric. Food Chem. 2001, 49(4), 1948-1951.
CrossRef - Gallagher, A. M., Flatt, P. R., Duffy, G. A. W. Y., Abdel-Wahab, Y. H. A. Nutr Res, 2003, 23(3), 413-424.
CrossRef - Edwards, C. A., Johnson, I. T., Read, N. W. Eur. J. Clin. Nutr. 1988, 42(4), 307-312.
CrossRef - Miller, G. L. Anal. Chem. 1959, 31(3), 426-428.
CrossRef - Denizot, F., Lang, R. J. Immunol. Methods. 1986, 89(2), 271-277.
CrossRef - Takigawa-Imamura, H., Sekine, T., Murata, M., Takayama, K., Nakazawa, K., Nakagawa, J. Biosci. Biotechnol. Biochem. 2003, 67(7), 1499-1506.
CrossRef - Oumaskour, K., Boujaber, N., Etahiri, S., Assobhei, O. Afr. J. Biotechnol. 2012, 11(104), 16831-16837.
- Richards, J. T., Kern, E. R., Glasgow, L. A., Overall Jr, J. C., Deign, E. F., Hatch, M. T. Antimicrob. Agents Chemother. 1978. 14(1), 24-30.
CrossRef - Athukorala, Y., Lee, K. W., Kim, S. K., Jeon, Y. J. Bioresour. Technol. 2007, 98(9), 1711-1716.
CrossRef - Abirami, R. G., Kowsalya, S. Int J Pharma Pharmaceut Sci. 2012, 4, 681-84.
- Boonchum, W., Peerapornpisal, Y., Kanjanapothi, D., Pekkoh, J., Amornlerdpison, D., Pumas, C. H. A. Y. A. K. O. R. N., Vacharapiyasophon, P. Int J Agric Biol. 2011, 13(1).
- Lopes, G., Sousa, C., Bernardo, J., Andrade, P. B., Valentão, P., Ferreres, F., Mouga, T. J. Phycol. 2011, 47(5), 1210-1218.
CrossRef - Yang, C. S., Brady, J. F., Hong, J. Y. FASEB J, 1992, 6(2), 737-744.
CrossRef - Thinakaran, T., Sivakumar, K. Int J Res Biol Sci. 2012, 2, 39-44.
- Latha, S., Daniel, M. J. Food Sci. Technol. 2001, 38(3), 272-273.
- Heo, S. J., Park, E. J., Lee, K. W., Jeon, Y. J. Bioresour. Technol. 2005, 96(14), 1613-1623.
CrossRef - Pérez, M. J., Falqué, E., Domínguez, H. Mar. Drugs. 2016, 14(3), 52.
CrossRef - Güven, K. C., Percot, A., Sezik, E. Mar. Drugs. 2010, 8(2), 269-284.
CrossRef - Ding Quan, L., Shui Chun, M., XiaoQing, Y., LiHua, F., XiaoPing, L. Heterocycles. 2012, 85(3), 661-666.
CrossRef - Gajalakshmi, D., Shettu, N., Murugesan, S. IJIRI. 2018, 6(2), 463-70.
- Gaysinski, M., Ortalo-Magné, A., Thomas, O. P., Culioli, G. Humana Press, New York, NY. 2015, 207-223
CrossRef - Asha Kanimozhi, S., Johnson, M., Renisheya Joy Jeba Malar, T. Int J Pharm Pharm Sci. 2012, 7(8), 393-397.
- Vishnu, K. M., Murugesan, S. World J Pharm Sci. 2014, 2(8), 817-820.
- Rajkumar, G., Bhavan, P. S., Suganya, M., Srinivasan, V., Karthik, M., and Udayasuriyan, R. Int Biol Biomed J. 2018, 4(1), 35-47.
- Syed, S., Arasu, A., Ponnuswamy, I. International Journal of Bio-Science and Bio-Technology 2015, 7(1), 185-190.
CrossRef - Song, Y., Manson, J. E., Buring, J. E., Sesso, H. D., Liu, S. J Am Coll Nutr. 2005, 24(5), 376-384.
CrossRef - Gupta, J., Gupta, A., Gupta, A. K. Int. J. Chem. Stud. 2016, 4(4), 190-198.
CrossRef - Aloud, A. A., Veeramani, C., Govindasamy, C., Alsaif, M. A., El Newehy, A. S., Al-Numair, K. S. Redox Rep. 2017, 22(6), 290-300.
CrossRef - Anantharaju, P. G., Gowda, P. C., Vimalambike, M. G., Madhunapantula, S. V. Nutr. 2016, 15(1), 1-16.
CrossRef - Asif, M. Chem. Int. 2015, 1(1), 35-52.
- Siangu, B. N., Sauda, S., John, M. K., Njue, W. M. Afr. J. Pure Appl. Chem. 2019, 13(3), 43-48.
- Adjimani, J. P., Asare, P. Toxicol. Rep. 2015, 2, 721-728.
CrossRef - ALENCAR, D. B., Diniz, J. C., Rocha, S. A., Pires-Cavalcante, K., Lima, R. L., Sousa, K. C., and Saker-Sampaio, S. An. Acad. Bras. Cienc. 2018, 90, 449-459.
CrossRef - Gunathilaka, T. L., Samarakoon, K. W., Ranasinghe, P., and Peiris, L. D. C. mol. 2019, 24(20), 3708.
CrossRef - Ravi, S., Banu, V. H., Nawas, P. M. A. methods. 2019, 11, 12.
- Li, K., Li, X. M., Gloer, J. B., Wang, B. G. Algal Res. 2021, 56, 102312.
CrossRef - Krishnan, B., Rathi, M. A., Nirmaladevi, N. Asian j. pharm. pharmacol. 2019, 5(5), 876-883.
CrossRef - Cojandaraj, L., Surya Prabha, U., Shyamala, M. E. Plant Arch. 2020, 20(2), 1075-1081.
- Rafique, R., Khan, K. M., Chigurupati, S., Wadood, A., Rehman, A. U., Salar, U., Perveen, S. Bioorg. Chem. 2020, 94, 103410.
CrossRef - Gong, L., Feng, D., Wang, T., Ren, Y., Liu, Y., Wang, J. Food Sci. Nutr. 2020, 8(12), 6320-6337.
CrossRef - Jaradat, N., Dacca, H., Hawash, M., Abualhasan, M. N. BMC Chem. 2021, 15(1), 1-13.
CrossRef - Salahuddin, M. A. H., Ismail, A., Kassim, N. K., Hamid, M., and Ali, M. S. M. Food Chem. 2020, 331, 127240.
CrossRef - Mechchate, H., Es-Safi, I., Louba, A., Alqahtani, A. S., Nasr, F. A., Noman, O. M., Bousta, D. mol. 2021, 26(2), 293.
CrossRef - Kang, G. G., Francis, N., Hill, R., Waters, D., Blanchard, C., Santhakumar, A. B. Int. J. Mol. Sci. 2020, 21(1), 140.
CrossRef - Jiang, Z., Yu, G., Liang, Y., Song, T., Zhu, Y., Ni, H., Oda, T. Biosci. Biotechnol. Biochem. 2019, 83(11), 2065-2074.
CrossRef - Naveen, J., Baskaran, R., Baskaran, V. Algal Res. 2021, 55, 102250.
CrossRef - Makkar, F., Chakraborty, K. Med Chem Res. 2018, 27(4), 1245-1259.
CrossRef - Kannan, S., Priya, V. V., Gayathri, R. Drug Invent. Today. 2019, 12(6).
CrossRef - Asif, A., Zeeshan, N., Mehmood, S. J. Food Biochem. 2020, 44(9), e13381.
CrossRef - Priyadarshini, S. R., Moses, J. A., Anandharamakrishnan, C. Nutr. Res. Rev. 2021, 1-27.
CrossRef - Vijayalakshmi, K., Selvaraj, C. I. Mol. Biol. Rep. 2018, 45(6), 2555-2561.
CrossRef - Kuruba, V., Gollapalli, P. Radiat Oncol J. 2018, 36(4), 265.
CrossRef - Pereira, R. M., Moura, L. P. D., Muñoz, V. R., Silva, A. S. R. D., Gaspar, R. S., Ropelle, E. R., Pauli, J. R. Mot. Rev. de Educ. Fis. 2017, 23.
CrossRef - Chadt, A., Al-Hasani, H. Pflugers Arch. 2020, 1-26.
This work is licensed under a Creative Commons Attribution 4.0 International License.